High density lipoprotein physiology
High Density Lipoprotein Microchapters |
Diagnosis |
---|
Treatment |
Clinical Trials |
Case Studies |
High density lipoprotein physiology On the Web |
American Roentgen Ray Society Images of High density lipoprotein physiology |
Risk calculators and risk factors for High density lipoprotein physiology |
Editor-In-Chief: C. Michael Gibson, M.S., M.D. [1]; Associate Editor(s)-in-Chief: Ayokunle Olubaniyi, M.B,B.S [2]
Overview
The physiological role of HDL centers around the reverse cholesterol transport system. Nascent HDL secreted into the plasma by the liver or intestine pick up free cholesterol from peripheral tissues and the arterial wall, an action mediated mainly by the ATP-binding cassette A1 (ABCA1). The enzyme lecithin-cholesteryl acetyltransferase (LCAT) catalyzes the esterification of the free cholesterol, and also converts the nascent HDL into the mature form. The esterified cholesterol is transported to the liver where cholesterylester transfer protein (CETP), an enzyme produced in the liver, acts on it transferring the cholesterol to other apo B containing lipoproteins. The cholesterol-deplete HDL gets broken down by triglyceride lipases releasing apo A-I which either takes up free cholesterol to continue the cycle, or gets eliminated in the kidneys. In addition to its atheroprotective against cardiovascular diseases, HDL also exhibits anti-oxidant, anti-inflammatory, anti-apoptotic, anti-coagulant, vasodilatory, and metabolic properties.
Physiology
Structure
Lipoprotein | Density | Size | % Protein | Cholesterol in Plasma | Triglyceride in Fasting Plasma | Major Apolipoprotein |
HDL | 1.063 - 1.210 g/mL | 6 - 10 mm | 40 - 55% | 0.9 - 1.6 mmol/L | 0.1 - 0.2 mmol/L | apoA-I, apoA-II |
For more information about the biochemistry of all lipoproteins, click here.
Shown below is a schematic image depicting the structure of the HDL. Note that the inner core is made of triglyceride and cholesterol esters whereas the surface is made of amphiphilic phospholipids along with apolipoproteins.
- HDL is the smallest of the lipoproteins. It is the densest because it contains the highest proportion of protein. It contains the A class of apolipoproteins. Apolipoprotein A-I is the main protein of HDL that removes excess cell cholesterol and protects against atherosclerosis.[1]
- The liver synthesizes these lipoproteins as complexes of apolipoproteins and phospholipids, which resemble cholesterol-free flattened spherical lipoprotein particles. They are capable of picking up cholesterol from cells they interact with.
- A plasma enzyme called lecithin-cholesterol acyltransferase (LCAT) converts the free cholesterol into cholesteryl ester (a more hydrophobic form of cholesterol) which is then sequestered into the core of the lipoprotein particle eventually making the newly synthesized HDL spherical. They increase in size as they circulate through the bloodstream and incorporate more cholesterol molecules into their structure.
- Thus it is the concentration of large HDL particles which more accurately reflects the HDL protective action, as opposed to the concentration of total HDL particles.[2] This ratio of large HDL to total HDL particles varies widely and is only measured by more sophisticated lipoprotein assays using either electrophoresis, originally developed in the 1970s, or newer nuclear magnetic resonance (NMR) spectroscopy which was developed in the 1990s.
- HDL particles are not inherently protective. It is only the HDL particles which become the largest (actually picking up and carrying cholesterol) that are protective. There is no reliable relationship between total HDL and large HDL, and more sophisticated analyses which actually measure large HDL, and not just total HDL, correlate much better with clinical outcomes.[3]
- Many studies have postulated an association between cholesterol efflux from peripheral tissue and Apo A-I HDL particles, whereas the HDL3 containing both Apo A-I and A-II are less effective.[4][5][6]
HDL Receptors
- ABCA1 transporter: it is expressed in the peripheral tissues, intestine, liver, and macrophages.[7] An increase in intracellular cholesterol content upregulates ABCA1 transporter which is responsible for cholesterol efflux from the intracellular pool.[8]
- ABCG1 transporter: it is expressed in the intestine and macrophages.[7] ABCG1 is also responsible for cholesterol efflux. In addition, ABCG1 may facilitate the oxidation of plasma membrane cholesterol domains.[9]
- Scavenger receptor class B type I (SR-BI): it is expressed in the liver, endothelial cells, and macrophages. It participates in reverse cholesterol transport in which extrahepatic cholesterol is delivered to the liver for excretion into the bile.[10][11] In macrophages it blunts cytokine production.[12] In endothelial cells it mediates HDL-induced endothelial nitric oxide synthase (eNOS) activation, proliferation, and migration.[13]
Enzymes Associated with HDL
Cholesterol Ester Transfer Protein (CETP)
- CETP mediates exchange of cholesterol between HDL particles, and triglyceride rich LDL and VLDL in both directions.
- CETP is normally present in both peripheral tissues and liver and functions to channel cholesterol to the liver for uptake and metabolism.
Lecithin-Cholesterol Acyltransferase (LCAT)
- LCAT is an enzyme that catalyzes the transfer of fatty acyl chain to free cholesterol which results in cholesteryl ester formation.[14]
- Its role in extracellular cholesterol metabolism may facilitate the uptake of cholesterol from peripheral tissues to liver into HDL particles by maintaining a concentration gradient for the efflux of free cholesterol which may play a major role in reverse cholesterol transport (RCT).[15]
Reverse Cholesterol Transport
HDL plays a pivotal role in cholesterol transport from peripheral tissues to the liver for excretion, a process known as reverse cholesterol transport. HDL’s protective atherosclerotic effect is related to its role in reverse cholesterol transport, where cholesterol efflux from macrophages to HDL is an important initial step.
Low concentration of HDL is one of the various risk factors of cardiovascular disease as demonstrated by preclinical and epidemiologic studies. Increasing HDL concentration by medical therapy, such as niacin and inhibition of cholesteryl ester transfer protein, was evaluated in many clinical trials. Studies such as ILLUMINATE,[16] dal-OUTCOMES,[17] and CHI-SQUARE, have failed to demonstrate an association between increasing HDL by therapy and improved cardiovascular outcomes. Higher cholesterol efflux capacity, however, is associated with a lower rate of cardiovascular disease, independently of HDL cholesterol concentration.[18] These findings highlight the importance of HDL function in reverse cholesterol transport and cholesterol efflux.

Adapted from Nature Reviews Drug Discovery. ABCA1= ATP-binding cassette transporter A1; ABCG1: ATP-binding cassette transporter G1; ABCG4: ATP-binding cassette transporter G4; ApoA-I= Apolipoprotein A-I; CETP: Cholesteryl transfer protein; LCAT: Lecithin cholesterol acyltransferase; SRBI: Scavenger receptor, class B, type I. [19]
Synthesis and Uptake of Cholesterol
- HDL consists of phospholipids and apolipoproteins, mainly apo A-I and/or apo A-II. Both the liver and the small intestine synthesize apo A-I while only the liver synthesizes apo A-II.
- Free apo A-I is released into the plasma as nascent HDLs. Nascent HDL readily takes up excess free cholesterol from the periphery such as fibroblasts, macrophages, and arterial wall, a process referred to as cholesterol efflux. This uptake of cholesterol is mediated by either ATP-binding cassette A1 (ABCA1), G1/G4, scavenger receptor class B type 1 (SR-B1), Cyp27A1, caveloin, and passive diffusion, leading to the formation of discoid HDL (a.k.a. pre-βHDL).
- Apo A-I is a cofactor of lecithin-cholesterol acetyltransferase (LCAT) which catalyzes the esterification of the free cholesterol bound to the discoid HDL. The apolipoprotein A1 acts as a signal protein in mobilizing cholesteryl esters from within the cells.

Maturation and Transfer of Cholesterol
- The esterified cholesterol moves into the hydrophobic core of the HDL, changing the HDL particle from discoid to spherical (mature HDL). This process also prevents the re-uptake of cholesterol by cells. LCAT is responsible for the maturation of HDL particles.
- The esterified cholesterol can be delivered back to the liver through a number of routes:
- CETP, secreted in the liver, transfers cholesterol from HDL to the apo B–containing lipoproteins e.g., very low-density lipoprotein (VLDL) or intermediate-density lipoprotein (IDL) to be taken up by the liver. Mutations of this transport protein gene causes familial HDL deficiencies and Tangier disease.
- HDL particles may be taken up directly by the liver.
- Free cholesterol may be taken up directly by the liver.
- HDL cholesterol esters may be selectively taken up via the scavenger receptor SR-B1, which is expressed in the liver.
Catabolism
- Triglyceride lipase degrades cholesterol-deplete HDL particles into small, dense HDL particles which release apo A-I (nascent HDL) after dissociation. The apo A-1 either rapidly re-uptakes free cholesterol again by ABCA1 to form discoid HDLs, or it is endocytosed into the kidney tubule, or cleared via glomerular filtration.
Role of HDL
Shown below is an image summarizing the physiologic functions of HDL in an acute and chronic setting. Please refer to the text below for details about each one of functions of HDL.
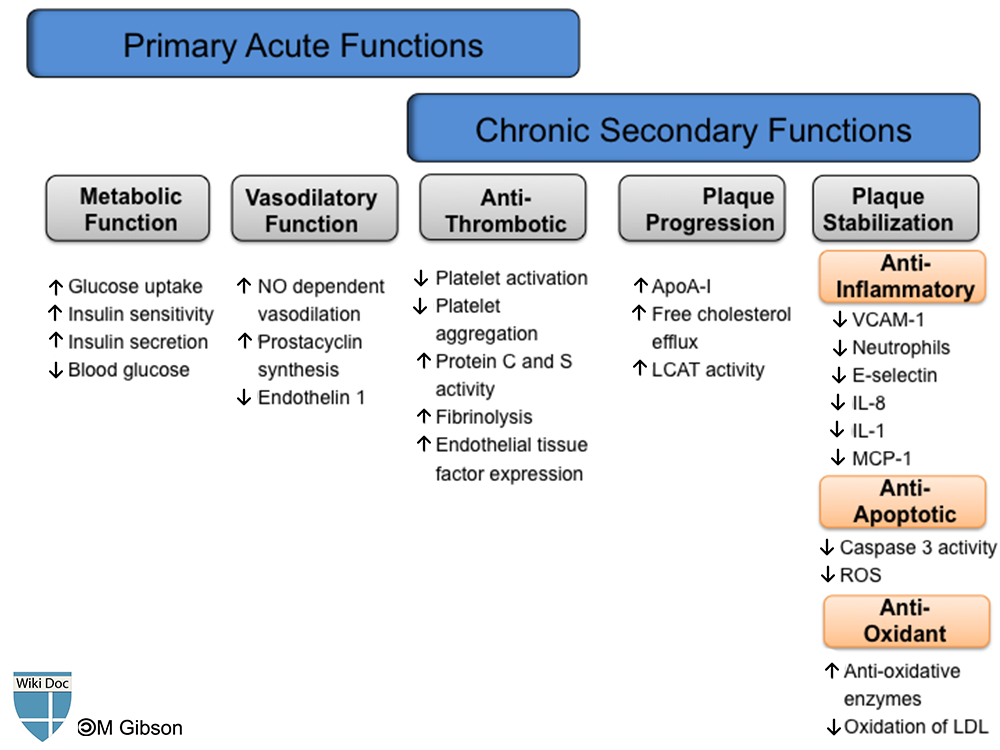
Atheroprotection
It has been established that HDL-cholesterol has an inverse correlation with future atherosclerotic cardiovascular complications. HDL and apo A-I exhibit many atheroprotective functions which primarily aim at removing cholesterol from peripheral tissues and the arterial wall through various efflux mechanisms, mainly the reverse cholesterol transport system. HDL also plays a role in the attenuation of plaque progression and promotion of plaque stabilization. These functions are exhibited through its anti-oxidative, anti-platelet, anti-apoptotic, and anti-inflammatory properties. With all these properties in context, HDL potentially protects against reperfusion ischemic injuries and secondary plaque rupture observed in post-acute coronary syndrome patients.
- Plasma HDL associated apolipoprotein M (apoM) modulates the ability of plasma to mobilize cellular cholesterol and protects against experimental atherosclerosis.[20]
- Animal models have demonstrated that the somatic gene transfer of human apo A-I can prevent the development of atherosclerosis or reverse preexisting atherosclerosis.[21][22][23][24]
- ATP-binding cassette (ABC) transporters ABCA1 and ABCG1 in endothelial cells and the scavenger receptor B type I mediate multiple intracellular signaling pathways as well as the efflux of cholesterol.[25]
Anti-Coagulation Function
HDL has the ability to inhibit platelet activation and aggregation by directly inhibiting platelet activation,[26][27] downregulating thromboxane A2 synthesis,[28] increasing the synthesis of prostacyclin,[29] and lowering the expression of the tissue factor which is required in the coagulation process.[30]
Anti-Oxidant Function
The formation of free oxygen radicals contributes to the pathogenesis and progression of atherosclerotic plaques. Oxidized LDL is engulfed by macrophages, which leads to further oxidation and production of foam cells. Oxidized LDL acts as a chemotactic agent for circulating monocytes, converts macrophages into foam cells, induces cytotoxic effects on the endothelium, inhibits motility of tissue macrophages, and stimulates the migration and proliferation of vascular smooth muscle cells.[31] HDL also inhibits the oxidative modification of oxidized LDLs,[32] and prevents their infiltration into the vessel wall.[33]
Anti-Inflammatory Function
HDL has anti-inflammatory functions in both endothelial cells and leukocytes. During inflammation, several leukocyte adhesion molecules are activated, which promotes the binding of leukocytes and formation of atheroma. HDL inhibits the activation of vascular cell adhesion molecule (VCAM-1),[34] interleukin-1-induced expresion of E-selectin,[35] interleukin-8, intracellular adhesion molecule (ICAM)-1, neutrophils,[36] monocytes,[37] and also prevents the binding of T-lymphocytes to monocytes thereby preventing the formation of pro-inflammatory cytokines.[38]
Metabolic Function
In a study on the effects and mechanisms of HDL on glucose metabolism, 13 type 2 diabetes patients were administered intravenous reconstituted HDL. There was a reduction in the plasma glucose of the patients due to an increase in plasma insulin in addition to the activation of AMP-activated protein kinase in the skeletal muscle. These findings suggest a role for HDL-raising therapies beyond atherosclerosis to address type 2 diabetes mellitus.[39]
Glucose Metabolism
HDL might modulate glucose homeostasis through several mechanisms such as the stimulation of insulin secretion,[40][41][39][42] enhancement of insulin sensitivity, and increased glucose uptake by skeletal muscle via activation of AMP-activated protein kinase (AMPK) signaling pathway.[43][41] Preliminary evidence from genetic engineering studies that manipulate expression of related genes such as ABCA1,[44][45][46][47] CETP,[48][49] ABCG1,[50] and apoA-I[43] suggests associations between plasma HDL concentrations and glycemic control. Silencing of microRNA species was also been associated with upregulation of these target genes along with elevation of functional HDL levels,[51][52][53][54] suggesting an extensively-linked yet fine-tuned state of homeostasis in energy metabolism.
Diabetes Mellitus
Type 2 diabetes mellitus and impaired fasting glucose are both associated with decreased levels of HDL.[55][56] In the Framingham Offspring Study, low levels of HDL cholesterol was reported as a significant predictor of incident type 2 diabetes mellitus.[57]
Anti-Apoptotic Function
Plasma HDL offers some cytoprotection against oxidized LDL-mediated apoptosis and generation of reactive oxygen species in-vitro .[58] HDL also protects endothelial cells from apoptosis and promotes their growth and their migration via SRBI-initiated signaling.[59] It is also suggested that the anti-apoptotic and proliferative effects of apoA-I are mediated through F1-ATPase-catalysed ADP production and subsequent P2Y13 receptor stimulation.[60]
Vasodilatory Function
HDL might play a role in the restoration of endothelial dysfunction implicated in the pathogenesis of type 2 diabetes. In one study, reconstituted HDL was infused in patients with type 2 diabetes and the vascular function (forearm blood flow) was assessed at 4 hours and 7 days post-infusion. HDL infusion was associated with an increase in the forearm blood flow in diabetic patients as compared to the controlled group, probably due to its effect on increasing the bioavailability of nitric oxide.[61]
References
- ↑ Mahler, DA.; Shuhart, CR.; Brew, E.; Stukel, TA. (1991). "Ventilatory responses and entrainment of breathing during rowing". Med Sci Sports Exerc. 23 (2): 186–92. PMID 2017014. Unknown parameter
|month=
ignored (help) - ↑ Kwiterovich PO. The Metabolic Pathways of High-Density Lipoprotein, Low-Density Lipoprotein, and Triglycerides: A Current Review. Am J Cardiol 2000;86(suppl):5L.
- ↑ Tran-Dinh A, Diallo D, Delbosc S; et al. (2013). "HDL and endothelial protection". British Journal of Pharmacology. doi:10.1111/bph.12174. PMID 23488589. Unknown parameter
|month=
ignored (help) - ↑ Yin K, Tang SL, Yu XH; et al. (2013). "Apolipoprotein A-I inhibits LPS-induced atherosclerosis in ApoE-/- mice possibly via activated STAT3-mediated upregulation of tristetraprolin". Acta Pharmacologica Sinica. doi:10.1038/aps.2013.10. PMID 23564081. Unknown parameter
|month=
ignored (help) - ↑ Mazer NA, Giulianini F, Paynter NP, Jordan P, Mora S (2013). "A Comparison of the Theoretical Relationship between HDL Size and the Ratio of HDL Cholesterol to Apolipoprotein A-I with Experimental Results from the Women's Health Study". Clinical Chemistry. doi:10.1373/clinchem.2012.196949. PMID 23426429. Unknown parameter
|month=
ignored (help) - ↑ Kappelle PJ, Gansevoort RT, Hillege HJ, Wolffenbuttel BH, Dullaart RP (2013). "Common variation in cholesteryl ester transfer protein: relationship of first major adverse cardiovascular events with the apolipoprotein B/apolipoprotein A-I ratio and the total cholesterol/high-density lipoprotein cholesterol ratio". Journal of Clinical Lipidology. 7 (1): 56–64. doi:10.1016/j.jacl.2012.05.003. PMID 23351584. Unknown parameter
|month=
ignored (help) - ↑ 7.0 7.1 Fitzgerald, ML.; Mujawar, Z.; Tamehiro, N. (2010). "ABC transporters, atherosclerosis and inflammation". Atherosclerosis. 211 (2): 361–70. doi:10.1016/j.atherosclerosis.2010.01.011. PMID 20138281. Unknown parameter
|month=
ignored (help) - ↑ Schwartz, K.; Lawn, RM.; Wade, DP. (2000). "ABC1 gene expression and ApoA-I-mediated cholesterol efflux are regulated by LXR". Biochem Biophys Res Commun. 274 (3): 794–802. doi:10.1006/bbrc.2000.3243. PMID 10924356. Unknown parameter
|month=
ignored (help) - ↑ Vaughan, AM.; Oram, JF. (2005). "ABCG1 redistributes cell cholesterol to domains removable by high density lipoprotein but not by lipid-depleted apolipoproteins". J Biol Chem. 280 (34): 30150–7. doi:10.1074/jbc.M505368200. PMID 15994327. Unknown parameter
|month=
ignored (help) - ↑ Brundert, M.; Ewert, A.; Heeren, J.; Behrendt, B.; Ramakrishnan, R.; Greten, H.; Merkel, M.; Rinninger, F. (2005). "Scavenger receptor class B type I mediates the selective uptake of high-density lipoprotein-associated cholesteryl ester by the liver in mice". Arterioscler Thromb Vasc Biol. 25 (1): 143–8. doi:10.1161/01.ATV.0000149381.16166.c6. PMID 15528479. Unknown parameter
|month=
ignored (help) - ↑ Out, R.; Hoekstra, M.; Spijkers, JA.; Kruijt, JK.; van Eck, M.; Bos, IS.; Twisk, J.; Van Berkel, TJ. (2004). "Scavenger receptor class B type I is solely responsible for the selective uptake of cholesteryl esters from HDL by the liver and the adrenals in mice". J Lipid Res. 45 (11): 2088–95. doi:10.1194/jlr.M400191-JLR200. PMID 15314100. Unknown parameter
|month=
ignored (help) - ↑ Guo, L.; Song, Z.; Li, M.; Wu, Q.; Wang, D.; Feng, H.; Bernard, P.; Daugherty, A.; Huang, B. (2009). "Scavenger Receptor BI Protects against Septic Death through Its Role in Modulating Inflammatory Response". J Biol Chem. 284 (30): 19826–34. doi:10.1074/jbc.M109.020933. PMID 19491399. Unknown parameter
|month=
ignored (help) - ↑ Besler, C.; Heinrich, K.; Rohrer, L.; Doerries, C.; Riwanto, M.; Shih, DM.; Chroni, A.; Yonekawa, K.; Stein, S. (2011). "Mechanisms underlying adverse effects of HDL on eNOS-activating pathways in patients with coronary artery disease". J Clin Invest. 121 (7): 2693–708. doi:10.1172/JCI42946. PMID 21701070. Unknown parameter
|month=
ignored (help) - ↑ Zechner, R.; Kostner, GM.; Dieplinger, H.; Degovics, G.; Laggner, P. (1984). "In vitro modification of the chemical composition of human plasma low density lipoproteins: effects of morphology and thermal properties". Chem Phys Lipids. 36 (2): 111–9. PMID 6532566. Unknown parameter
|month=
ignored (help) - ↑ Ohashi, R.; Mu, H.; Wang, X.; Yao, Q.; Chen, C. (2005). "Reverse cholesterol transport and cholesterol efflux in atherosclerosis". QJM. 98 (12): 845–56. doi:10.1093/qjmed/hci136. PMID 16258026. Unknown parameter
|month=
ignored (help) - ↑ Barter PJ, Caulfield M, Eriksson M, Grundy SM, Kastelein JJ, Komajda M; et al. (2007). "Effects of torcetrapib in patients at high risk for coronary events". N Engl J Med. 357 (21): 2109–22. doi:10.1056/NEJMoa0706628. PMID 17984165.
- ↑ Schwartz GG, Olsson AG, Abt M, Ballantyne CM, Barter PJ, Brumm J; et al. (2012). "Effects of dalcetrapib in patients with a recent acute coronary syndrome". N Engl J Med. 367 (22): 2089–99. doi:10.1056/NEJMoa1206797. PMID 23126252.
- ↑ Rohatgi A, Khera A, Berry JD, Givens EG, Ayers CR, Wedin KE; et al. (2014). "HDL Cholesterol Efflux Capacity and Incident Cardiovascular Events". N Engl J Med. doi:10.1056/NEJMoa1409065. PMID 25404125.
- ↑ Linsel-Nitschke P, Tall AR (2005). "HDL as a target in the treatment of atherosclerotic cardiovascular disease". Nat Rev Drug Discov. 4 (3): 193–205. doi:10.1038/nrd1658. PMID 15738977.
- ↑ Elsøe S, Christoffersen C, Luchoomun J, Turner S, Nielsen LB (2013). "Apolipoprotein M promotes mobilization of cellular cholesterol in vivo". Biochim Biophys Acta. 1831 (7): 1287–92. PMID 24046869.
- ↑ Benoit P, Emmanuel F, Caillaud JM, Bassinet L, Castro G, Gallix P; et al. (1999). "Somatic gene transfer of human ApoA-I inhibits atherosclerosis progression in mouse models". Circulation. 99 (1): 105–10. PMID 9884386.
- ↑ Tangirala RK, Tsukamoto K, Chun SH, Usher D, Puré E, Rader DJ (1999). "Regression of atherosclerosis induced by liver-directed gene transfer of apolipoprotein A-I in mice". Circulation. 100 (17): 1816–22. PMID 10534470.
- ↑ Navab M, Anantharamaiah GM, Hama S, Garber DW, Chaddha M, Hough G; et al. (2002). "Oral administration of an Apo A-I mimetic Peptide synthesized from D-amino acids dramatically reduces atherosclerosis in mice independent of plasma cholesterol". Circulation. 105 (3): 290–2. PMID 11804981.
- ↑ Ma J, Liao XL, Lou B, Wu MP (2004). "Role of apolipoprotein A-I in protecting against endotoxin toxicity". Acta Biochim Biophys Sin (Shanghai). 36 (6): 419–24. PMID 15188057.
- ↑ Prosser HC, Ng MK, Bursill CA (2012). "The role of cholesterol efflux in mechanisms of endothelial protection by HDL". Curr Opin Lipidol. 23 (3): 182–9. doi:10.1097/MOL.0b013e328352c4dd. PMID 22488423.
- ↑ Calkin, AC.; Drew, BG.; Ono, A.; Duffy, SJ.; Gordon, MV.; Schoenwaelder, SM.; Sviridov, D.; Cooper, ME.; Kingwell, BA. (2009). "Reconstituted high-density lipoprotein attenuates platelet function in individuals with type 2 diabetes mellitus by promoting cholesterol efflux". Circulation. 120 (21): 2095–104. doi:10.1161/CIRCULATIONAHA.109.870709. PMID 19901191. Unknown parameter
|month=
ignored (help) - ↑ Lerch, PG.; Spycher, MO.; Doran, JE. (1998). "Reconstituted high density lipoprotein (rHDL) modulates platelet activity in vitro and ex vivo". Thromb Haemost. 80 (2): 316–20. PMID 9716159. Unknown parameter
|month=
ignored (help) - ↑ Brill, A.; Fuchs, TA.; Chauhan, AK.; Yang, JJ.; De Meyer, SF.; Köllnberger, M.; Wakefield, TW.; Lämmle, B.; Massberg, S. (2011). "von Willebrand factor-mediated platelet adhesion is critical for deep vein thrombosis in mouse models". Blood. 117 (4): 1400–7. doi:10.1182/blood-2010-05-287623. PMID 20959603. Unknown parameter
|month=
ignored (help) - ↑ Fleisher, LN.; Tall, AR.; Witte, LD.; Miller, RW.; Cannon, PJ. (1982). "Stimulation of arterial endothelial cell prostacyclin synthesis by high density lipoproteins". J Biol Chem. 257 (12): 6653–5. PMID 7045092. Unknown parameter
|month=
ignored (help) - ↑ Viswambharan, H.; Ming, XF.; Zhu, S.; Hubsch, A.; Lerch, P.; Vergères, G.; Rusconi, S.; Yang, Z. (2004). "Reconstituted high-density lipoprotein inhibits thrombin-induced endothelial tissue factor expression through inhibition of RhoA and stimulation of phosphatidylinositol 3-kinase but not Akt/endothelial nitric oxide synthase". Circ Res. 94 (7): 918–25. doi:10.1161/01.RES.0000124302.20396.B7. PMID 14988229. Unknown parameter
|month=
ignored (help) - ↑ Kita T, Kume N, Minami M, Hayashida K, Murayama T, Sano H; et al. (2001). "Role of oxidized LDL in atherosclerosis". Ann N Y Acad Sci. 947: 199–205, discussion 205-6. PMID 11795267.
- ↑ Parthasarathy S, Barnett J, Fong LG (1990). "High-density lipoprotein inhibits the oxidative modification of low-density lipoprotein". Biochim Biophys Acta. 1044 (2): 275–83. PMID 2344447.
- ↑ Galle, J.; Ochslen, M.; Schollmeyer, P.; Wanner, C. (1994). "Oxidized lipoproteins inhibit endothelium-dependent vasodilation. Effects of pressure and high-density lipoprotein". Hypertension. 23 (5): 556–64. PMID 8175161. Unknown parameter
|month=
ignored (help) - ↑ Dimayuga, P.; Zhu, J.; Oguchi, S.; Chyu, KY.; Xu, XO.; Yano, J.; Shah, PK.; Nilsson, J.; Cercek, B. (1999). "Reconstituted HDL containing human apolipoprotein A-1 reduces VCAM-1 expression and neointima formation following periadventitial cuff-induced carotid injury in apoE null mice". Biochem Biophys Res Commun. 264 (2): 465–8. doi:10.1006/bbrc.1999.1278. PMID 10529386. Unknown parameter
|month=
ignored (help) - ↑ Cockerill, GW.; Huehns, TY.; Weerasinghe, A.; Stocker, C.; Lerch, PG.; Miller, NE.; Haskard, DO. (2001). "Elevation of plasma high-density lipoprotein concentration reduces interleukin-1-induced expression of E-selectin in an in vivo model of acute inflammation". Circulation. 103 (1): 108–12. PMID 11136694. Unknown parameter
|month=
ignored (help) - ↑ Murphy, AJ.; Woollard, KJ.; Suhartoyo, A.; Stirzaker, RA.; Shaw, J.; Sviridov, D.; Chin-Dusting, JP. (2011). "Neutrophil activation is attenuated by high-density lipoprotein and apolipoprotein A-I in in vitro and in vivo models of inflammation". Arterioscler Thromb Vasc Biol. 31 (6): 1333–41. doi:10.1161/ATVBAHA.111.226258. PMID 21474825. Unknown parameter
|month=
ignored (help) - ↑ Murphy, AJ.; Woollard, KJ.; Hoang, A.; Mukhamedova, N.; Stirzaker, RA.; McCormick, SP.; Remaley, AT.; Sviridov, D.; Chin-Dusting, J. (2008). "High-density lipoprotein reduces the human monocyte inflammatory response". Arterioscler Thromb Vasc Biol. 28 (11): 2071–7. doi:10.1161/ATVBAHA.108.168690. PMID 18617650. Unknown parameter
|month=
ignored (help) - ↑ Carpintero, R.; Gruaz, L.; Brandt, KJ.; Scanu, A.; Faille, D.; Combes, V.; Grau, GE.; Burger, D. (2010). "HDL interfere with the binding of T cell microparticles to human monocytes to inhibit pro-inflammatory cytokine production". PLoS One. 5 (7): e11869. doi:10.1371/journal.pone.0011869. PMID 20686620.
- ↑ 39.0 39.1 Drew, BG.; Duffy, SJ.; Formosa, MF.; Natoli, AK.; Henstridge, DC.; Penfold, SA.; Thomas, WG.; Mukhamedova, N.; de Courten, B. (2009). "High-density lipoprotein modulates glucose metabolism in patients with type 2 diabetes mellitus". Circulation. 119 (15): 2103–11. doi:10.1161/CIRCULATIONAHA.108.843219. PMID 19349317. Unknown parameter
|month=
ignored (help) - ↑ Brunham, LR.; Kruit, JK.; Pape, TD.; Timmins, JM.; Reuwer, AQ.; Vasanji, Z.; Marsh, BJ.; Rodrigues, B.; Johnson, JD. (2007). "Beta-cell ABCA1 influences insulin secretion, glucose homeostasis and response to thiazolidinedione treatment". Nat Med. 13 (3): 340–7. doi:10.1038/nm1546. PMID 17322896. Unknown parameter
|month=
ignored (help) - ↑ 41.0 41.1 Abderrahmani, A.; Niederhauser, G.; Favre, D.; Abdelli, S.; Ferdaoussi, M.; Yang, JY.; Regazzi, R.; Widmann, C.; Waeber, G. (2007). "Human high-density lipoprotein particles prevent activation of the JNK pathway induced by human oxidised low-density lipoprotein particles in pancreatic beta cells". Diabetologia. 50 (6): 1304–14. doi:10.1007/s00125-007-0642-z. PMID 17437081. Unknown parameter
|month=
ignored (help) - ↑ Fryirs, MA.; Barter, PJ.; Appavoo, M.; Tuch, BE.; Tabet, F.; Heather, AK.; Rye, KA. (2010). "Effects of high-density lipoproteins on pancreatic beta-cell insulin secretion". Arterioscler Thromb Vasc Biol. 30 (8): 1642–8. doi:10.1161/ATVBAHA.110.207373. PMID 20466975. Unknown parameter
|month=
ignored (help) - ↑ 43.0 43.1 Han, R.; Lai, R.; Ding, Q.; Wang, Z.; Luo, X.; Zhang, Y.; Cui, G.; He, J.; Liu, W. (2007). "Apolipoprotein A-I stimulates AMP-activated protein kinase and improves glucose metabolism". Diabetologia. 50 (9): 1960–8. doi:10.1007/s00125-007-0752-7. PMID 17639303. Unknown parameter
|month=
ignored (help) - ↑ Vergeer, M.; Brunham, LR.; Koetsveld, J.; Kruit, JK.; Verchere, CB.; Kastelein, JJ.; Hayden, MR.; Stroes, ES. (2010). "Carriers of loss-of-function mutations in ABCA1 display pancreatic beta-cell dysfunction". Diabetes Care. 33 (4): 869–74. doi:10.2337/dc09-1562. PMID 20067955. Unknown parameter
|month=
ignored (help) - ↑ Daimon, M.; Kido, T.; Baba, M.; Oizumi, T.; Jimbu, Y.; Kameda, W.; Yamaguchi, H.; Ohnuma, H.; Tominaga, M. (2005). "Association of the ABCA1 gene polymorphisms with type 2 DM in a Japanese population". Biochem Biophys Res Commun. 329 (1): 205–10. doi:10.1016/j.bbrc.2005.01.119. PMID 15721294. Unknown parameter
|month=
ignored (help) - ↑ Villarreal-Molina, MT.; Flores-Dorantes, MT.; Arellano-Campos, O.; Villalobos-Comparan, M.; Rodríguez-Cruz, M.; Miliar-García, A.; Huertas-Vazquez, A.; Menjivar, M.; Romero-Hidalgo, S. (2008). "Association of the ATP-binding cassette transporter A1 R230C variant with early-onset type 2 diabetes in a Mexican population". Diabetes. 57 (2): 509–13. doi:10.2337/db07-0484. PMID 18003760. Unknown parameter
|month=
ignored (help) - ↑ Koseki, M.; Matsuyama, A.; Nakatani, K.; Inagaki, M.; Nakaoka, H.; Kawase, R.; Yuasa-Kawase, M.; Tsubakio-Yamamoto, K.; Masuda, D. (2009). "Impaired insulin secretion in four Tangier disease patients with ABCA1 mutations". J Atheroscler Thromb. 16 (3): 292–6. PMID 19556721. Unknown parameter
|month=
ignored (help) - ↑ López-Ríos, L.; Pérez-Jiménez, P.; Martínez-Quintana, E.; Rodriguez González, G.; Díaz-Chico, BN.; Nóvoa, FJ.; Serra-Majem, L.; Chirino, R. (2011). "Association of Taq 1B CETP polymorphism with insulin and HOMA levels in the population of the Canary Islands". Nutr Metab Cardiovasc Dis. 21 (1): 18–24. doi:10.1016/j.numecd.2009.06.009. PMID 19822408. Unknown parameter
|month=
ignored (help) - ↑ Zhong, S.; Sharp, DS.; Grove, JS.; Bruce, C.; Yano, K.; Curb, JD.; Tall, AR. (1996). "Increased coronary heart disease in Japanese-American men with mutation in the cholesteryl ester transfer protein gene despite increased HDL levels". J Clin Invest. 97 (12): 2917–23. doi:10.1172/JCI118751. PMID 8675707. Unknown parameter
|month=
ignored (help) - ↑ Sturek, JM.; Castle, JD.; Trace, AP.; Page, LC.; Castle, AM.; Evans-Molina, C.; Parks, JS.; Mirmira, RG.; Hedrick, CC. (2010). "An intracellular role for ABCG1-mediated cholesterol transport in the regulated secretory pathway of mouse pancreatic beta cells". J Clin Invest. 120 (7): 2575–89. doi:10.1172/JCI41280. PMID 20530872. Unknown parameter
|month=
ignored (help) - ↑ Rayner, KJ.; Suárez, Y.; Dávalos, A.; Parathath, S.; Fitzgerald, ML.; Tamehiro, N.; Fisher, EA.; Moore, KJ.; Fernández-Hernando, C. (2010). "MiR-33 contributes to the regulation of cholesterol homeostasis". Science. 328 (5985): 1570–3. doi:10.1126/science.1189862. PMID 20466885. Unknown parameter
|month=
ignored (help) - ↑ Marquart, TJ.; Allen, RM.; Ory, DS.; Baldán, A. (2010). "miR-33 links SREBP-2 induction to repression of sterol transporters". Proc Natl Acad Sci U S A. 107 (27): 12228–32. doi:10.1073/pnas.1005191107. PMID 20566875. Unknown parameter
|month=
ignored (help) - ↑ Najafi-Shoushtari, SH.; Kristo, F.; Li, Y.; Shioda, T.; Cohen, DE.; Gerszten, RE.; Näär, AM. (2010). "MicroRNA-33 and the SREBP host genes cooperate to control cholesterol homeostasis". Science. 328 (5985): 1566–9. doi:10.1126/science.1189123. PMID 20466882. Unknown parameter
|month=
ignored (help) - ↑ Wang, MD.; Franklin, V.; Sundaram, M.; Kiss, RS.; Ho, K.; Gallant, M.; Marcel, YL. (2007). "Differential regulation of ATP binding cassette protein A1 expression and ApoA-I lipidation by Niemann-Pick type C1 in murine hepatocytes and macrophages". J Biol Chem. 282 (31): 22525–33. doi:10.1074/jbc.M700326200. PMID 17553802. Unknown parameter
|month=
ignored (help) - ↑ Gatti, A.; Maranghi, M.; Bacci, S.; Carallo, C.; Gnasso, A.; Mandosi, E.; Fallarino, M.; Morano, S.; Trischitta, V. (2009). "Poor glycemic control is an independent risk factor for low HDL cholesterol in patients with type 2 diabetes". Diabetes Care. 32 (8): 1550–2. doi:10.2337/dc09-0256. PMID 19487641. Unknown parameter
|month=
ignored (help) - ↑ Drexel, H.; Aczel, S.; Marte, T.; Benzer, W.; Langer, P.; Moll, W.; Saely, CH. (2005). "Is atherosclerosis in diabetes and impaired fasting glucose driven by elevated LDL cholesterol or by decreased HDL cholesterol?". Diabetes Care. 28 (1): 101–7. PMID 15616241. Unknown parameter
|month=
ignored (help) - ↑ Wilson, PW.; Meigs, JB.; Sullivan, L.; Fox, CS.; Nathan, DM.; D'Agostino, RB. (2007). "Prediction of incident diabetes mellitus in middle-aged adults: the Framingham Offspring Study". Arch Intern Med. 167 (10): 1068–74. doi:10.1001/archinte.167.10.1068. PMID 17533210. Unknown parameter
|month=
ignored (help) - ↑ de Souza, JA.; Vindis, C.; Nègre-Salvayre, A.; Rye, KA.; Couturier, M.; Therond, P.; Chantepie, S.; Salvayre, R.; Chapman, MJ. (2010). "Small, dense HDL 3 particles attenuate apoptosis in endothelial cells: pivotal role of apolipoprotein A-I". J Cell Mol Med. 14 (3): 608–20. doi:10.1111/j.1582-4934.2009.00713.x. PMID 19243471. Unknown parameter
|month=
ignored (help) - ↑ Saddar S, Mineo C, Shaul PW (2010). "Signaling by the high-affinity HDL receptor scavenger receptor B type I." Arterioscler Thromb Vasc Biol. 30 (2): 144–50. doi:10.1161/ATVBAHA.109.196170. PMID 20089950.
- ↑ Radojkovic C, Genoux A, Pons V, Combes G, de Jonge H, Champagne E; et al. (2009). "Stimulation of cell surface F1-ATPase activity by apolipoprotein A-I inhibits endothelial cell apoptosis and promotes proliferation". Arterioscler Thromb Vasc Biol. 29 (7): 1125–30. doi:10.1161/ATVBAHA.109.187997. PMID 19372457.
- ↑ van Etten, RW.; de Koning, EJ.; Verhaar, MC.; Gaillard, CA.; Rabelink, TJ. (2002). "Impaired NO-dependent vasodilation in patients with Type II (non-insulin-dependent) diabetes mellitus is restored by acute administration of folate". Diabetologia. 45 (7): 1004–10. doi:10.1007/s00125-002-0862-1. PMID 12136399. Unknown parameter
|month=
ignored (help)