Malaria: Difference between revisions
No edit summary |
No edit summary |
||
Line 1: | Line 1: | ||
{{CMG}} | {{CMG}} | ||
'''For patient information click [[{{PAGENAME}} (patient information)|here]]''' | '''For patient information click [[{{PAGENAME}} (patient information)|here]]''' | ||
{{Infobox_Disease | {{Infobox_Disease | ||
Line 26: | Line 18: | ||
}} | }} | ||
{{InfectiousDisease | |||
= | |description=Malaria overview | ||
|historicalPerspective=Malaria_historical_perspective | |||
= | |pathophysiology=Malaria_pathophysiology | ||
|epidemiology=Malaria_epidemiology_and_demographics | |||
|causedby=Malaria_causes | |||
}} | |||
==References== | ==References== | ||
<div class="references-small" style="-moz-column-count:2; column-count:2;"> | <div class="references-small" style="-moz-column-count:2; column-count:2;"> |
Revision as of 14:20, 14 June 2012
Editor-In-Chief: C. Michael Gibson, M.S., M.D. [4] For patient information click here
Malaria | ||
![]() | ||
---|---|---|
Plasmodium falciparum ring-forms and gametocytes in human blood. | ||
ICD-10 | B50 | |
ICD-9 | 084 | |
DiseasesDB | 7728 | |
MedlinePlus | 000621 | |
MeSH | C03.752.250.552 |
{{#meta: itemprop="medicalWebPageAudiences" content="patient"}}{{#meta: itemprop="medicalWebPageSpecialities" content="cardiology"}}{{#meta: itemprop="medicalWebPageInfoTypes" content="symptoms,diagnosis,treatment,causes,prognosis,complications"}} [[Natural Progression::{{{naturalProgression}}}| ]] Classification Classic::Classification Atypical::
Overview
https://https://www.youtube.com/watch?v=2O3YrdUZQ5U%7C350}} |
Malaria Microchapters |
Diagnosis |
---|
Treatment |
Case studies |
Malaria On the Web |
American Roentgen Ray Society Images of Malaria |
Editor-In-Chief: C. Michael Gibson, M.S., M.D. [5]; Associate Editor(s)-in-Chief: Usama Talib, BSc, MD [6]
Overview
Malaria is a vector-borne infectious disease caused by protozoan parasites. Malaria is one of the most common infectious diseases and an enormous public-health problem. The disease is caused by protozoan parasites of the genus Plasmodium. The most serious forms of the disease are caused by Plasmodium falciparum and Plasmodium vivax, but other related species (Plasmodium ovale, Plasmodium malariae, and sometimes Plasmodium knowlesi) can also infect humans. This group of human-pathogenic Plasmodium species is usually referred to as malaria parasites.
Historical Perspective
Malaria is considered one of the oldest infections known to mankind. The symptoms of malaria were first believed to be caused by noxious elements.[1] It was not until 1880 when Charles Louis Alphone Laveran discovered the Plasmodium parasite in blood smears of patients with malaria.[2] The role of mosquitos in the transmission of malaria to humans was discovered a few years later. Consequently, the entire life cycle of the Plasmodium parasite remained enigmatic until 1982.[3] Although malaria has always been treated using quinine, an alkaloid derived from barks of cinchona, the first synthetic quinine was produced in 1948.[4][5] In 2014, the first candidate for anti-malarial vaccine was developed.
Pathophysiology
Malaria in humans develops via two phases: an exoerythrocytic (hepatic) and an erythrocytic phase. When an infected mosquito pierces a person's skin to take a blood meal, sporozoites in the mosquito's saliva enter the bloodstream and migrate to the liver.[6]
Classification
The classification of malaria can be made according to the strains of Plasmodium species. There are 5 common Plasmodium species that infect humans: P. falciparum, P. ovale, P. vivax, P. malariae, and P. knowlesi. Malaria can also be classified according to severity of infection: uncomplicated vs. severe.[6]
Causes
Malaria is a vector-borne infectious disease caused by protozoan parasites. P. vivax is the most common cause of infection, responsible for about 80 % of all malaria cases. However, P. falciparum is the most important cause of disease, and responsible for about 15% of infections and 90% of deaths.[7]
Epidemiology and Demographics
Worldwide, 3.4 billion people live in areas at risk of malaria transmission in 106 countries and territories. The World Health Organization estimates that in 2012 malaria caused 207 million clinical episodes, and 627,000 deaths. An estimated 91% of deaths in 2010 were in the African Region.[8] The vast majority of cases of malaria occur in children under the age of 5 years.[9] Malaria is presently endemic in a broad band around the equator, in areas of the Americas, many parts of Asia, and much of Africa; however, it is in sub-Saharan Africa where 85– 90% of malaria fatalities occur.[10] Where malaria is found depends mainly on climatic factors such as temperature, humidity, and rainfall.
Risk Factors
Travel to endemic areas is a risk factor for malaria. For travelers, regions associated with the highest estimated relative risk of infection are West Africa and Oceania. Human behavior, often dictated by socioeconomic situations, can influence the risk of malaria for individuals and communities. In addition, children and pregnant women are at a higher risk of contracting malaria. Certain biologic characteristics can protect against particular types of malaria. Two genetic factors, the sickle cell trait and absence of Duffy blood group, have been shown to be epidemiologically significant.[11]
Screening
Screening of malaria is important in Sub-Saharan refugees and blood donors.[12]
Natural History, Complications ad Prognosis
Following the infective bite of the Anopheles mosquito, a period of time goes by before the first symptoms appear, with the incubation period varying between 7 to 30 days depending on the immune status of the patient, type and strain of the plasmodium, the dose of sporozoites injected on the bite, and the presence of prophylactic drugs. The classical but rarely observed malaria attack lasts 6-10 hours, and it consists of a cold stage, hot stage, and sweating stage. Severe malaria is almost exclusively caused by P. falciparum infections and usually arises 6-14 days following infection.[13] Complications of severe malaria include splenomegaly, severe headache, cerebral ischemia, hepatomegaly, hypotension, ARDS, and hemoglobinuria with renal failure. Severe malaria can progress extremely rapidly and cause death within hours or days. In the most severe cases, fatality rates can exceed 20%, even with intensive care and treatment.[14][15]
Diagnosis
Symptoms
The hallmark symptom of malaria is fever, which commonly occurs in paroxysms, separated by fever-free time intervals. The classical but rarely observed malaria attack lasts 6-10 hours, and it consists of a cold stage, hot stage, and sweating stage. Other common symptoms of malaria include chills, headache, nausea, vomiting, weakness, night sweats, flu-like symptoms, and myalgia. In the presence of a paroxysmal fever, travel history to a country where malaria is endemic is an important alert for the diagnosis.[16] Most importantly, malaria symptoms must be distinguished as to whether they reflect an uncomplicated or a severe course of infection. While uncomplicated infection is a benign process, severe malaria causes organ damage and is considered a medical emergency.
Physical Examination
Physical findings in malaria may include fever, weakness, pallor, jaundice, and perspiration. Other findings on physical exam are tachycardia, tachypnea, splenomegaly, and hepatomegaly.
Laboratory Findings
The diagnosis of malaria is confirmed by the identification of the malaria parasite in the patient's blood under microscopy. Laboratory tests may also reveal anemia with decreased hemoglobin, hematocrit, and haptoglobin in addition to either a decreased or increased leukocyte count. Biochemistry may reveal hypoglycemia, elevated LDH, acidosis, and mild hyponatremia due to vomiting and diarrhea. In severe disease, elevated creatinine, proteinuria, and hemoglobinuria may be present secondary to renal failure.[12][17] Other malaria-specific tests include: the antigen detection test, PCR, and serology test for the presence of antibodies against the plasmodium. Drug resistance tests should also be performed to assess the susceptibility of the microorganism to the antimalarial drugs.
X ray
X rays are not required for the diagnosis of malaria.
Ultrasound
Ultrasound is not required for the diagnosis of malaria. It can be used to assess splenomegaly or complications of malaria.[15]
CT scan
CT scan is not required for the diagnosis of malaria.
MRI
MRI is not required for the diagnosis of malaria.
Other diagnostic studies
Malaria is diagnosed by complementing the clinical findings of the patient with malaria-specific diagnostic studies. Other diagnostic studies like PCR are being used to help with malaria elimination.[18]
Treatment
Medical Therapy
Active malaria infection with P. falciparum is a medical emergency requiring hospitalization. Infection with P. vivax, P. ovale or P. malariae can often be treated on an outpatient basis. Treatment of malaria involves supportive measures as well as specific antimalarial drugs. When properly treated, someone with malaria can expect a complete cure.[19]
Primary Prevention
Although some are under development, no vaccine is currently available for malaria. RTS,S malaria vaccine has been proposed in July 2014 and is pending approval by the European Medicines Agency (EMA). Other more recent vaccine, such as PfSPZ malaria vaccine is currently being studied for clinical efficacy.
Preventative drugs must be taken continuously to reduce the risk of infection. Most adults from endemic areas have a degree of long-term recurrent infection and also of partial resistance; the resistance reduces with time and adults may become susceptible to severe malaria if they have spent a significant amount of time in non-endemic areas. Individuals are strongly recommended to take full precautions if they return to an endemic region.
Secondary Prevention
Malaria transmission can be reduced by preventing mosquito bites with mosquito nets and insect repellents, or by mosquito control measures such as spraying insecticides inside houses and draining standing water where mosquitoes lay their eggs.
Cost-Effectiveness of Therapy
Although effective anti-malarial drugs are on the market, the disease remains a threat to people living in endemic areas who have no proper and prompt access to effective drugs. Access to pharmacies and health facilities, as well as drug costs, are major obstacles. Médecins Sans Frontières estimates that the cost of treating a malaria-infected person in an endemic country was between US $0.25 and $2.40 per dose in 2002.[20]
Future or Investigational Therapies
RTS,S is considered the first anti-malaria vaccine candidate. The phase 3 trial for the vaccine involving young African children demonstrated significant efficacy beyond the efficacy observed by the use of conventional preventative measures, proving further benefit in preventing malaria. On July 24 2014, GSK submitted a regulatory application to the European Medicines Agency (EMA) for approval of the vaccine. If granted, the World Health Organization (WHO) intends to recommend the RTS,S malaria vaccine in 2015. Other vaccines are also in the making, but still require further validation of their clinical efficacy.
References
- ↑ Cox FE (2010). "History of the discovery of the malaria parasites and their vectors". Parasit Vectors. 3 (1): 5. doi:10.1186/1756-3305-3-5. PMC 2825508. PMID 20205846.
- ↑ Laveran CL (1982). "Classics in infectious diseases: A newly discovered parasite in the blood of patients suffering from malaria. Parasitic etiology of attacks of malaria: Charles Louis Alphonse Laveran (1845-1922)". Rev Infect Dis. 4 (4): 908–11. PMID 6750753.
- ↑ Krotoski WA, Collins WE, Bray RS, Garnham PC, Cogswell FB, Gwadz RW; et al. (1982). "Demonstration of hypnozoites in sporozoite-transmitted Plasmodium vivax infection". Am J Trop Med Hyg. 31 (6): 1291–3. PMID 6816080.
- ↑ Seeman JI (2007). "The Woodward-Doering/Rabe-Kindler total synthesis of quinine: setting the record straight". Angew Chem Int Ed Engl. 46 (9): 1378–413. doi:10.1002/anie.200601551. PMID 17294412.
- ↑ Kaufman TS, Rúveda EA (2005). "The quest for quinine: those who won the battles and those who won the war". Angew Chem Int Ed Engl. 44 (6): 854–85. doi:10.1002/anie.200400663. PMID 15669029.
- ↑ 6.0 6.1 Long CA, Zavala F (2017). "Immune Responses in Malaria". Cold Spring Harb Perspect Med. doi:10.1101/cshperspect.a025577. PMID 28389518.
- ↑ Mendis K, Sina B, Marchesini P, Carter R (2001). "The neglected burden of Plasmodium vivax malaria" (PDF). Am J Trop Med Hyg. 64 (1-2 Suppl): 97–106. PMID 11425182.
- ↑ Malaria Facts. CDC.gov accessed on 07/24/2014 [1]
- ↑ Greenwood BM, Bojang K, Whitty CJ, Targett GA (2005). "Malaria". Lancet. 365: 1487–1498. PMID 15850634.
- ↑ Layne SP. "Principles of Infectious Disease Epidemiology /" (PDF). EPI 220. UCLA Department of Epidemiology. Retrieved 2007-06-15.
- ↑ Factors. CDC.gov Accessed on 7/24/2014
- ↑ 12.0 12.1 "Immigrant and Refugee Health".
- ↑ Trampuz A, Jereb M, Muzlovic I, Prabhu R (2003). "Clinical review: Severe malaria". Crit Care. 7 (4): 315–23. PMID 12930555.
- ↑ Kain K, Harrington M, Tennyson S, Keystone J (1998). "Imported malaria: prospective analysis of problems in diagnosis and management". Clin Infect Dis. 27 (1): 142–9. PMID 9675468.
- ↑ 15.0 15.1 Unger HW, Ome-Kaius M, Karl S, Singirok D, Siba P, Walker J; et al. (2015). "Factors associated with ultrasound-aided detection of suboptimal fetal growth in a malaria-endemic area in Papua New Guinea". BMC Pregnancy Childbirth. 15: 83. doi:10.1186/s12884-015-0511-6. PMC 4404558. PMID 25881316.
- ↑ Mandell, Gerald (2010). Mandell, Douglas, and Bennett's principles and practice of infectious diseases. Philadelphia, PA: Churchill Livingstone/Elsevier. ISBN 0443068399.
- ↑ Mandell, Gerald (2010). Mandell, Douglas, and Bennett's principles and practice of infectious diseases. Philadelphia, PA: Churchill Livingstone/Elsevier. ISBN 0443068399.
- ↑ Srisutham S, Saralamba N, Malleret B, Rénia L, Dondorp AM, Imwong M (2017). "Four human Plasmodium species quantification using droplet digital PCR". PLoS One. 12 (4): e0175771. doi:10.1371/journal.pone.0175771. PMID 28423028.
- ↑ If I get malaria, will I have it for the rest of my life? CDC publication, Accessed 14 Nov 2006
- ↑ Medecins Sans Frontieres, "What is the Cost and Who Will Pay?"
Historical Perspective
Malaria Microchapters |
Diagnosis |
---|
Treatment |
Case studies |
Malaria On the Web |
American Roentgen Ray Society Images of Malaria |
Editor-In-Chief: C. Michael Gibson, M.S., M.D. [7]; Associate Editor(s)-In-Chief: Yazan Daaboul, Serge Korjian, Alison Leibowitz [8], Marjan Khan M.B.B.S.[9]
Overview
The symptoms of malaria, one of the oldest known infections, were initially believed to be caused by noxious elements. In 1880 Charles Louis Alphone Laveran discovered the Plasmodium parasite in blood smears of patients with malaria. The role of mosquitos in the transmission of malaria to humans was discovered a few years later. The entire life cycle of the Plasmodium parasite remained enigmatic until 1982.Although malaria has historically been treated using quinine, an alkaloid derived from barks of cinchona, the first synthetic quinine was produced in 1948.In 2014, the first candidate for anti-malarial vaccine was developed.
Historical Perspective
Discovery
Ancient History
- Malaria is one of the earliest discovered global diseases, which continues to infect hundreds of million people worldwide. Frequently, it has been regarded as the most significant disease over the past three thousand years. Since antiquity, the malarial syndrome has been described in ancient China, India, Greece, and Egypt.[1]
- Hippocrates, Homer, and other Greek and Roman physicians often referred to malaria as the “marsh fever”, “intermittent fever”, and “ague”.[1][2]
- The name “malaria” was only coined in the mid-eighteenth century, derived from two Latin words that collectively translate to “bad air”.[3]
Discovery and Differentiation of the Malaria Parasite
- Malaria was originally believed to be an airborne noxious element or miasma from swamps. It was not until 1880, when Charles Louis Alphone Laveran, a French military physician, discovered an infectious parasite when he microscopically examined blood smears of 44 malaria patients and “noticed among the red corpuscles elements that seemed to be parasites”.[4]
- Fourteen years later in 1897, Sir Ronald Ross, an Indian-born British bacteriologist, isolated malarial oocysts in Anopheles mosquitos and was able to prove that the culcine mosquito is the malarial vector for avian malaria.[5]
- Consequently, he also won the Nobel Prize for Physiology or Medicine in 1902 for his research on malarial transmission and life cycle.[3]
- Ross’s discovery was then followed by a similar discovery one year later for Anopheles mosquito and human malaria by Italian researchers Giovanni Battista Grassi, Amico Bignami, Giuseppe Bastianelli, Angelo Celli, Camillo Golgi, and Ettore Marchiafava.[1]
Outbreaks
Malaria in the United States
- Malaria was mostly eliminated from the United States in the early 1950s.[6]
- Between 1957 and 2011, in the United States, 63 outbreaks of locally transmitted mosquito-borne malaria occurred. In such outbreaks, local mosquitoes become infected by biting individuals carrying malaria parasites (acquired in endemic areas), subsequently transmitting malaria to local residents.[6]
- Between 1963 and 2011, 97 cases of transfusion-transmitted malaria were reported in the United States. Approximately two thirds of these cases could have been prevented if the implicated donors had been deferred according to established guidelines.[6]
Landmark Events in the Development of Treatment Strategies
Synthetic Quinine
- Robert Woodward and William vonEggers Doering developed the total synthesis of quinine in 1944.
- Paul Rabe and Karl Kindler’s report on converting d-quinotoxine into quinine in 1918.[7][8]
- Originally, quinine is an alkaloid derived from barks of cinchona and Remijia tree species that were proven to be effective in the treatment of malaria.
- With Woodward and Doering’s discovery of the first artificial quinine, the first synthetic pharmacological agent to treat malaria was produced.[8]
Chloroquine
- In 1934, chloroquine (Resochin) was synthesized followed by Sontochin.[9]
- These compounds belonged to a new class of antimalarials known as four-amino quinolines.
- Following World War II, chloroquine emerged as the principal weapons in the WHO's ambitious “global eradication” malaria campaign.
- Chloroquine-resistant P. falciparum (CRPF) probably arose de novo from four independent geographic locations:
- The Thai-Cambodian border around 1957.
- Venezuela and the nearby Magdalena Valley of Colombia around 1960.
- Port Moresby, Papua New Guinea, in the mid-1970s.
- In Africa, CRPF was first found in 1978, spreading next to inland coastal areas and by 1983, to Sudan, Uganda, Zambia, and Malawi.
Sulfadoxine-Pyrimethamine
- Sulfadoxine-pyrimethamine (SP),the most widely used antifolate antimalarial combination today, was introduced in Thailand in 1967. Resistance to SP was reported in Thailand later that year.[9]
- The pyrimidine derivative, proguanil, emerged from the antimalarial pipeline during World War II. it stimulated further study for making agents that block folate synthesis in parasites and bacteria, and resulted in the development of pyrimethamine.
- It became apparent that malaria parasites could quickly alter the target enzyme of the two drugs, leading to resistance.
- sulfonamides were then combined with proguanil or pyrimethamine for increasing efficacy, and forestalling or preventing the development of resistance.
Mefloquine
- Mefloquine was a collaborative achievement of the U.S. Army Medical Research and Development Command, the World Health Organization (WHO/TDR), and Hoffman-La Roche.[9]
- Mefloquine's efficacy in preventing falciparum malaria was acknowledged in 1974.
- Mefloquine resistance began to appear in Asia around the time of the drug's availability in 1985.
Artemisinin
- Artemisinin is the antimalarial isolated by Chinese scientists in 1972 from Artemisia annua (sweet wormwood).[9]
- The earliest report of its use appears in a Chinese book found in the Mawanhgolui Han dynasty tombs dating to 168 BC.
- Artemisinin and other Artemether-group drugs are the main line of treatment against drug-resistant malaria in many areas of southeast Asia.
- The number of Artemisinin-based combination therapy treatment courses procured from manufacturers increased globally from 187 million in 2010 to 409 million in 2016.[10]
- Artemisinin partial resistance likely emerged prior to 2001.To date, it has been confirmed in 5 countries: Cambodia, the Lao People’s Democratic Republic, Myanmar, Thailand and Viet Nam.[10]
Partial Eradication of Malaria
- Upon the understanding of malaria's mode of transmission and mechanisms of disease, mosquito control and prompt diagnosis and treatment, allowed most European countries to eliminate malaria before the Second World War.[11]
- In 1955, the Global Malaria Eradication Program was established in an effort to control and eliminate malaria, as well as to reduce the malarial burden in regions of moderate prevalence outside tropical Africa.
- The financial coverage and expertise to fight malaria further expanded to include global efforts, such as "Global Fund to Fight HIV, TB, and Malaria", "U.S. President's Malaria Initiative", and "World Bank's Booster Program".[11]
- In 2008, the World Health Organization (WHO) announced a multibillion-dollar initiative to eradicate malaria, partially funded by international donors.[12]
- In 2005, with a grant funding from the Bill and Melinda Gates Foundation, PATH Malaria Vaccine Initiative (MVI), a non-profit organization, collaborated with Glaxosmithkline, to develop an anti-malarial vaccine. The vaccine has been administered, alongside other infant vaccines, through the Expanded Program on Immunization (EPI).
- In 2011, the first co-primary end point from the phase 3 trial of RTS, S/AS01 malaria vaccine was published, followed by a second co-primary end point in 2012.[13]
- The vaccine was used to protect against uncomplicated and severe malaria in infants. In July 2014, Glaxosmithkline applied for approval to be the world's first anti-malarial vaccine. Other malarial vaccines are currently being developed, but still require further validation of their clinical efficacy.
References
- ↑ 1.0 1.1 1.2 1.3 Cox FE (2010). "History of the discovery of the malaria parasites and their vectors". Parasit Vectors. 3 (1): 5. doi:10.1186/1756-3305-3-5. PMC 2825508. PMID 20205846.
- ↑ Bruce-Chwatt LJ (1981). "Alphonse Laveran's discovery 100 years ago and today's global fight against malaria". J R Soc Med. 74 (7): 531–6. PMC 1439072. PMID 7021827.
- ↑ 3.0 3.1 3.2 Dutta HM, Dutt AK (1978). "Malarial ecology: a global perspective". Soc Sci Med. 12 (2D): 69–84. PMID 81525.
- ↑ Laveran CL (1982). "Classics in infectious diseases: A newly discovered parasite in the blood of patients suffering from malaria. Parasitic etiology of attacks of malaria: Charles Louis Alphonse Laveran (1845-1922)". Rev Infect Dis. 4 (4): 908–11. PMID 6750753.
- ↑ Ross R (1897). "Observations on a Condition Necessary to the Transformation of the Malaria Crescent". Br Med J. 1 (1883): 251–5. PMC 2432879. PMID 20756775.
- ↑ 6.0 6.1 6.2 Malaria Facts. CDC.gov accessed on 07/24/2014 [2]
- ↑ Seeman JI (2007). "The Woodward-Doering/Rabe-Kindler total synthesis of quinine: setting the record straight". Angew Chem Int Ed Engl. 46 (9): 1378–413. doi:10.1002/anie.200601551. PMID 17294412.
- ↑ 8.0 8.1 Kaufman TS, Rúveda EA (2005). "The quest for quinine: those who won the battles and those who won the war". Angew Chem Int Ed Engl. 44 (6): 854–85. doi:10.1002/anie.200400663. PMID 15669029.
- ↑ 9.0 9.1 9.2 9.3 "A Brief History of Malaria - Saving Lives, Buying Time - NCBI Bookshelf".
- ↑ 10.0 10.1 "WHO | Q&A on artemisinin resistance, SYSTEM DO NOT MOVE OR EDIT".
- ↑ 11.0 11.1 Mendis K, Rietveld A, Warsame M, Bosman A, Greenwood B, Wernsdorfer WH (2009). "From malaria control to eradication: The WHO perspective". Trop Med Int Health. 14 (7): 802–9. doi:10.1111/j.1365-3156.2009.02287.x. PMID 19497083.
- ↑ Okie S (2008). "A new attack on malaria". N Engl J Med. 358 (23): 2425–8. doi:10.1056/NEJMp0803483. PMID 18525039.
- ↑ RTS,S Clinical Trials Partnership. Agnandji ST, Lell B, Fernandes JF, Abossolo BP, Methogo BG; et al. (2012). "A phase 3 trial of RTS,S/AS01 malaria vaccine in African infants". N Engl J Med. 367 (24): 2284–95. doi:10.1056/NEJMoa1208394. PMID 23136909.
Pathophysiology
https://https://www.youtube.com/watch?v=2O3YrdUZQ5U%7C350}} |
Malaria Microchapters |
Diagnosis |
---|
Treatment |
Case studies |
Malaria On the Web |
American Roentgen Ray Society Images of Malaria |
Editor-In-Chief: C. Michael Gibson, M.S., M.D. [10]; Associate Editor(s)-In-Chief: Yazan Daaboul, Serge Korjian, Alison Leibowitz [11] , Marjan Khan M.B.B.S.[12]
Overview
Malaria in humans develops in two phases: an exo-erythrocytic (hepatic) and an erythrocytic phase. When an infected mosquito pierces an individual's skin for blood, sporozoites in the mosquito's saliva enter the bloodstream and subsequently migrate to the liver.
Pathophysiology
- Malaria is caused by protozoan parasites of the genus Plasmodium (phylum Apicomplexa).
- In humans, malaria is caused by P. falciparum, P. malariae, P. ovale, P. vivax and Plasmodium knowlesi.
- P. vivax is the most common cause of infection, responsible for about 80% of all malaria cases. P. falciparum, the most significant cause of disease, is responsible for about 15% of infections and 90% of deaths.[1][2]
Life Cycle
- The life cycle of Plasmodium parasites begin when the sporozoite, a haploid form of the parasite, is injected into the human bloodstream by the female Anopheles mosquito.[3]
- The sporozoites travel in the bloodstream to the liver and invade human hepatocytes. Over 1-2 weeks later, in the exo-erythrocytic phase, the sporozoites grow into schizonts and produce thousands of merozoites in each hepatocyte.
- The merozoite is a haploid form of the parasite.[3] While some hepatocytes rupture and release the merozoites, other parasites remain dormant within the liver.[3] The release of merozoites, by the hepatocytes, into the bloodsteam results in the manifestation of the malarial symptoms.
- The latency of cell rupture between various hepatocytes and the consequent merozoite release into the bloodstream, is responsible for the characteristic periodic fever associated with malarial infections.[3]
- As merozoites are released into the bloodstream, they infect erythrocytes and undergo asexual multiplication (mitosis). Some merozoites continue the cycle of asexual replication into mature trophozoites and schizonts, which rupture to re-release merozoites. Others develop into sexual forms, the gametocytes, which involve male (microgametocyte) and female (macrogametocyte) parasites.[3]
- Though biting, Anopheles mosquitos ingest the gametocytes within the red blood cells, initiating the sporogonic cycle inside the mosquito. In the mosquito's gut, the cells burst and the gametocytes are then released, allowing their development into mature gametes.
- The fusion of male and female gametes forms diploid zygotes, which become ookinetes, motile and elongated forms of the parasites. Within the mosquito midgut wall, they develop into oocysts.[3]
- As oocysts continue to grow, they divide into active haploid forms, the sporozoites. Thousands of sporozoites are produced in each oocyst. When oocysts burst following 1-2 weeks, sporozoites travel to the mosquito's salivary glands, so that when the mosquito bites other humans they inject the sporozoites into their bloodstream, leading the cycle to restart.[3]
- Some species, such as P. vivax and P. ovale are characterized by their ability to produce hypnozoites, an intermediate stage where the parasite remains dormant for a few months/years before reactivation into merozoites. The hypnozoite stage allows teh species to demonstrate late relapses and long incubation periods.[4]

Human Factors
- Some human factors may provide a protective advantage against malarial infection. Individuals with sickle cell trait, defined as the heterozygous for the abnormal globin gene, HbS, are protected against P. falciparum.
- In individuals with sickle cell trait, red blood cells invaded by P. falciparum tend to sickle more readily than other red blood cells, leading them to be eliminated from the bloodstream by macrophages.[5]
- The preventative advantage demonstrated in heterozygous sickle cell patients is not observed in patients who have sickle cell anemia and carry a homozygous sickle gene. Contrarily, these patients are more susceptible to lethal complications of severe anemia.[5]
- Other similar hematological diseases that provide a protective effect against malaria are thalassemia, hemoglobin C, and G6PD deficiency.
- Individuals who have a negative Duffy blood group are resistant to infection by P. vivax. These individuals are still susceptible to other species of malaria, especially P. ovale, which frequently infects individuals with negative Duffy blood group.[6]
- P. falciparum infection have higher mean parasitaemia index, P. vivax infection generally exhibit low parasitaemia index due to its preference to invade reticulocytes rather than erythrocytes.[7]
Associated Conditions
Severe malarial anaemia
- Severe malarial anaemia is defined as a haemoglobin concentration of <5 g/dL and the presence of high parasitaemia >10,000 parasites/μl.[8]
- The proposed mechanisms involved in severe malaria anaemia is a cumulative of loss of RBCs due to infection, lysis of uninfected RBCs in the circulation, and impaired RBC production.[9]
- In P. vivax infections, ~34 uninfected RBCs are removed for every infected RBCs in the circulation whereas in P.falciparum infections, about 8 uninfected RBCs are lysed for every infected RBC.[10]
Acute respiratory distress syndrome (ARDS)
- This condition is associated with deep breathing, respiratory distress, pulmonary oedema, airway obstruction, impaired function of the alveoli and decreased gas exchange.[11]
- Autopsy studies in ARDS cases from P. vivax prior to antimalarial treatment has showed heavy infiltrates of intravascular mononuclear cells, endothelial and alveolar damages, and absence of parasite sequestration in the pulmonary vasculature.[12]
- Study from Brazil has reported an infiltration of neutrophils in alveolar capillaries even after parasites were cleared from peripheral blood by antimalarial drug treatment.[13]
Pregnancy-associated malaria
- Pregnants are more susceptible to malaria infections because of their somewhat compromised immune status, especially during the first and second trimesters of pregnancy.[14]
- P. falciparum parasite has the ability to massively sequester in the placenta.[15]
- The sequestration of parasite-infected RBCs in the intervillous space of placenta and the adherence of infected RBCs to the syncytiotrophoblast cell layer are the contributors to pregnancy associated malaria pathogenesis.[16]
- The wide spread clinical conditions resulting from pregnancy associated malaria are severe anemia, intrauterine growth retardation, low birth weight, preterm delivery, miscarriage, perinatal mortality, and death in the mother.[17]
References
- ↑ Mendis K, Sina B, Marchesini P, Carter R (2001). "The neglected burden of Plasmodium vivax malaria" (PDF). Am J Trop Med Hyg. 64 (1-2 Suppl): 97–106. PMID 11425182.
- ↑ Long CA, Zavala F (2017). "Immune Responses in Malaria". Cold Spring Harb Perspect Med. doi:10.1101/cshperspect.a025577. PMID 28389518.
- ↑ 3.0 3.1 3.2 3.3 3.4 3.5 3.6 "Malaria". National Institute of Allergy and Infectious Diseases. NIH. Apr. 3 2012. Retrieved Jul 24 2014. Check date values in:
|accessdate=, |date=
(help) - ↑ Cogswell F (1992). "The hypnozoite and relapse in primate malaria". Clin Microbiol Rev. 5 (1): 26–35. PMID 1735093.
- ↑ 5.0 5.1 Luzzatto L (2012). "Sickle cell anaemia and malaria". Mediterr J Hematol Infect Dis. 4 (1): e2012065. doi:10.4084/MJHID.2012.065. PMC 3499995. PMID 23170194.
- ↑ "Malaria". Centers for Disease Control and Prevention. CDC. Nov 9 2012. Retrieved Jul 24 2014. Check date values in:
|accessdate=, |date=
(help) - ↑ Mueller I, Galinski MR, Baird JK, Carlton JM, Kochar DK, Alonso PL, del Portillo HA (September 2009). "Key gaps in the knowledge of Plasmodium vivax, a neglected human malaria parasite". Lancet Infect Dis. 9 (9): 555–66. doi:10.1016/S1473-3099(09)70177-X. PMID 19695492.
- ↑ Lamikanra AA, Brown D, Potocnik A, Casals-Pascual C, Langhorne J, Roberts DJ (July 2007). "Malarial anemia: of mice and men". Blood. 110 (1): 18–28. doi:10.1182/blood-2006-09-018069. PMID 17341664.
- ↑ Anstey NM, Russell B, Yeo TW, Price RN (May 2009). "The pathophysiology of vivax malaria". Trends Parasitol. 25 (5): 220–7. doi:10.1016/j.pt.2009.02.003. PMID 19349210.
- ↑ Price RN, Simpson JA, Nosten F, Luxemburger C, Hkirjaroen L, ter Kuile F, Chongsuphajaisiddhi T, White NJ (November 2001). "Factors contributing to anemia after uncomplicated falciparum malaria". Am. J. Trop. Med. Hyg. 65 (5): 614–22. PMC 4337986. PMID 11716124.
- ↑ Douglas NM, Anstey NM, Buffet PA, Poespoprodjo JR, Yeo TW, White NJ, Price RN (April 2012). "The anaemia of Plasmodium vivax malaria". Malar. J. 11: 135. doi:10.1186/1475-2875-11-135. PMC 3438072. PMID 22540175.
- ↑ Valecha N, Pinto RG, Turner GD, Kumar A, Rodrigues S, Dubhashi NG, Rodrigues E, Banaulikar SS, Singh R, Dash AP, Baird JK (November 2009). "Histopathology of fatal respiratory distress caused by Plasmodium vivax malaria". Am. J. Trop. Med. Hyg. 81 (5): 758–62. doi:10.4269/ajtmh.2009.09-0348. PMID 19861606.
- ↑ Lacerda MV, Fragoso SC, Alecrim MG, Alexandre MA, Magalhães BM, Siqueira AM, Ferreira LC, Araújo JR, Mourão MP, Ferrer M, Castillo P, Martin-Jaular L, Fernandez-Becerra C, del Portillo H, Ordi J, Alonso PL, Bassat Q (October 2012). "Postmortem characterization of patients with clinical diagnosis of Plasmodium vivax malaria: to what extent does this parasite kill?". Clin. Infect. Dis. 55 (8): e67–74. doi:10.1093/cid/cis615. PMID 22772803.
- ↑ Rogerson SJ, Mwapasa V, Meshnick SR (December 2007). "Malaria in pregnancy: linking immunity and pathogenesis to prevention". Am. J. Trop. Med. Hyg. 77 (6 Suppl): 14–22. PMID 18165470.
- ↑ Beeson JG, Duffy PE (2005). "The immunology and pathogenesis of malaria during pregnancy". Curr. Top. Microbiol. Immunol. 297: 187–227. PMID 16265906.
- ↑ Rogerson SJ, Hviid L, Duffy PE, Leke RF, Taylor DW (February 2007). "Malaria in pregnancy: pathogenesis and immunity". Lancet Infect Dis. 7 (2): 105–17. doi:10.1016/S1473-3099(07)70022-1. PMID 17251081.
- ↑ Souza RM, Ataíde R, Dombrowski JG, Ippólito V, Aitken EH, Valle SN, Álvarez JM, Epiphanio S, Epiphânio S, Marinho CR (2013). "Placental histopathological changes associated with Plasmodium vivax infection during pregnancy". PLoS Negl Trop Dis. 7 (2): e2071. doi:10.1371/journal.pntd.0002071. PMC 3573078. PMID 23459254.
Epidemiology and Demographics
Malaria Microchapters |
Diagnosis |
---|
Treatment |
Case studies |
Malaria On the Web |
American Roentgen Ray Society Images of Malaria |
Editor-In-Chief: C. Michael Gibson, M.S., M.D. [13]; Associate Editor(s)-in-Chief: Rim Halaby, M.D. [14]
Overview
Worldwide, 3.4 billion people live in areas at risk of malaria transmission in 106 countries and territories. The World Health Organization estimates that in 2012 malaria caused 207 million clinical episodes, and 627,000 deaths. An estimated 91% of deaths in 2010 occurred in the African Region. The vast majority of cases of malaria occur in children under the age of 5 years. Malaria is presently endemic in a broad band around the equator, in areas of the Americas, many parts of Asia, and much of Africa; however, it is in sub-Saharan Africa where 85– 90% of malaria fatalities occur. Malaria is present depending primarily on climatic factors such as temperature, humidity, and rainfall.
Epidemiology and Demographics
P. vivax is the most common cause of infection, responsible for about 80 % of all malaria cases. However, P. falciparum is the most important cause of disease, and responsible for about 15% of infections and 90% of deaths.[1]
Incidence
- The World Health Organization estimates that in 2012 malaria caused 207 million clinical episodes worldwide.[2]
- In the United States, approximately 1,500–2,000 cases of malaria are reported every year, almost all in recent travelers. Reported malaria cases reached a 40-year high of 1,925 in 2011.[2]
Age
The vast majority of cases of malaria occurs in children under the age of 5 years.[3]
Mortality
- The World Health Organization estimates that in 2012 malaria caused 207 million clinical episodes, and 627,000 deaths. An estimated 91% of deaths in 2010 were in the African Region.[2]
Geographic Distribution
- Malaria is presently endemic in a broad band around the equator, in areas of the Americas, many parts of Asia, and much of Africa; however, it is in sub-Saharan Africa where 85– 90% of malaria fatalities occur.[4]
- The geographic distribution of malaria within large regions is complex, and malarial and malaria-free areas are often found close to each other.[5]
- Shown below is an image depicting an approximation of the parts of the world where malaria transmission occurs (source: CDC).
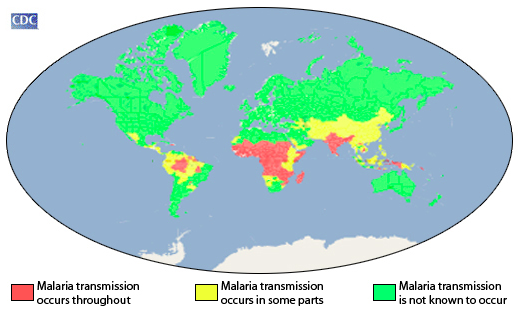
Rural Areas vs Cities
- Malaria is more common in rural areas than in cities; this contrasts with dengue fever, where urban areas present the greater risk.[7]
- The cities of the Vietnam, Laos and Cambodia are essentially malaria-free, but the disease is present in many rural regions.[8]
- In Africa malaria is present in both rural and urban areas, though the risk is lower in the larger cities.[9]
Climatic Factors
- Malaria is found mainly on climatic factors such as temperature, humidity, and rainfall.
- Temperature is particularly critical. For example, at temperatures below 20°C (68°F), Plasmodium falciparum (which causes severe malaria) cannot complete its growth cycle in the Anopheles mosquito, and thus cannot be transmitted.
- In many malaria-endemic countries, malaria transmission does not occur in all parts of the country. Even within tropical and subtropical areas, transmission will not occur:
- At very high altitudes
- During colder seasons in some areas
- In deserts (excluding the oases)
- In some countries where transmission has been interrupted through successful control/elimination programs.
- Generally, in warmer regions closer to the equator transmission will be more intense and malaria is transmitted year-round. The highest transmission is found in Africa South of the Sahara and in parts of Oceania such as Papua New Guinea.
- In cooler regions, transmission will be less intense and more seasonal. There, P. vivax might be more prevalent because it tolerates better lower temperatures.
- Many temperate areas, such as western Europe and the United States, economic development and public health measures have succeeded in eliminating malaria. However, most of these areas have Anopheles mosquitoes that can transmit malaria, and the reintroduction of the disease is a constant risk.
- In drier areas, outbreaks of malaria can be predicted with reasonable accuracy by mapping rainfall.[10]
Socio-Economic Effects
- Malaria is not just a disease commonly associated with poverty, but is also a cause of poverty and a major hindrance to economic development.
- The disease has been associated with major negative economic effects on regions where it is widespread.
- A comparison of average per capita GDP in 1995, adjusted to give parity of purchasing power, between malarious and non-malarious countries demonstrates a fivefold difference (US$1,526 versus US$8,268).
- In countries where malaria is common, average per capita GDP has risen (between 1965 and 1990) only 0.4% per year, compared to 2.4% per year in other countries.[11]
- Correlation does not imply causation, and the prevalence is at least partly because these regions do not have the financial capacities to prevent malaria. In its entirety, the economic impact of malaria has been estimated to cost Africa US$12 billion every year.
- The economic impact includes costs of health care, working days lost due to sickness, days lost in education, decreased productivity due to brain damage from cerebral malaria, and loss of investment and tourism.[3]
- In some countries with a heavy malaria burden, the disease may account for as much as 40% of public health expenditure, 30-50% of inpatient admissions, and up to 50% of outpatient visits.[12]
References
- ↑ Mendis K, Sina B, Marchesini P, Carter R (2001). "The neglected burden of Plasmodium vivax malaria" (PDF). Am J Trop Med Hyg. 64 (1-2 Suppl): 97–106. PMID 11425182.
- ↑ 2.0 2.1 2.2 Malaria Facts. CDC.gov accessed on 07/24/2014 [3]
- ↑ 3.0 3.1 Greenwood BM, Bojang K, Whitty CJ, Targett GA (2005). "Malaria". Lancet. 365: 1487–1498. PMID 15850634.
- ↑ Layne SP. "Principles of Infectious Disease Epidemiology /" (PDF). EPI 220. UCLA Department of Epidemiology. Retrieved 2007-06-15.
- ↑ Greenwood B, Mutabingwa T (2002). "Malaria in 2002". Nature. 415: 670–2. PMID 11832954.
- ↑ "Centers for Disease Control and Prevention (CDC)".
- ↑ Van Benthem B, Vanwambeke S, Khantikul N, Burghoorn-Maas C, Panart K, Oskam L, Lambin E, Somboon P (2005). "Spatial patterns of and risk factors for seropositivity for dengue infection". Am J Trop Med Hyg. 72 (2): 201–8. PMID 15741558.
- ↑ Trung H, Van Bortel W, Sochantha T, Keokenchanh K, Quang N, Cong L, Coosemans M (2004). "Malaria transmission and major malaria vectors in different geographical areas of Southeast Asia". Trop Med Int Health. 9 (2): 230–7. PMID 15040560.
- ↑ Keiser J, Utzinger J, Caldas de Castro M, Smith T, Tanner M, Singer B (2004). "Urbanization in sub-saharan Africa and implication for malaria control". Am J Trop Med Hyg. 71 (2 Suppl): 118–27. PMID 15331827.
- ↑ Grover-Kopec E, Kawano M, Klaver R, Blumenthal B, Ceccato P, Connor S (2005). "An online operational rainfall-monitoring resource for epidemic malaria early warning systems in Africa". Malar J. 4: 6. PMID 15663795.
- ↑ Sachs J, Malaney P (2002). "The economic and social burden of malaria". Nature. 415: 680–5. PMID 11832956.
- ↑ Roll Back Malaria. "Economic costs of malaria". WHO. Retrieved 2006-09-21.
Causes
Malaria Microchapters |
Diagnosis |
---|
Treatment |
Case studies |
Malaria On the Web |
American Roentgen Ray Society Images of Malaria |
Editor-In-Chief: C. Michael Gibson, M.S., M.D. [15]
Overview
- A plasmodium is also the macroscopic form of the protist known as a slime mould.
Plasmodium is a genus of parasitic protozoa. Infection with this genus is known as malaria. The parasite always has two hosts in its life cycle: a mosquito vector and a vertebrate host. At least ten species infect humans. Other species infect other animals, including birds, reptiles and rodents.
Taxonomy and host range
The genus Plasmodium was created in 1885 by Marchiafava and Celli and there are over 175 species currently recognized. New species continue to be described. [1]
The genus is currently (2006) in need of reorganization as it has been shown that parasites belonging to the genera Haemocystis and Hepatocystis appear to be closely related to Plasmodium. It is likely that other species such as Haemoproteus meleagridis will be included in this genus once it is revised.
Host range among the mammalian orders is non uniform. At least 29 species infect non human primates; rodents outside the tropical parts of Africa are rarely affected; a few species are known to infect bats, porcupines and squirrels; carnivores, insectivores and marsupials are not known to act as hosts.
In 1898 Ronald Ross demonstrated the existence of Plasmodium in the wall of the midgut and salivary glands of a Culex mosquito. For this discovery he won the Nobel Prize in 1902. However credit must also be given to the Italian professor Giovanni Battista Grassi, who showed that human malaria could only be transmitted by Anopheles mosquitoes. It is worth noting, however, that for some species the vector may not be a mosquito.
Mosquitoes of the genera Culex, Anopheles, Culiceta, Mansonia and Aedes may act as vectors. The currently known vectors for human malaria (> 100 species) all belong to the genus Anopheles. Bird malaria is commonly carried by species belonging to the genus Culex. Only female mosquitoes bite. Aside from blood both sexes live on nectar, but one or more blood meals are needed by the female for egg laying as the protein content of nectar is very low. The life cycle of Plasmodium was discovered by Ross who worked with species from the genus Culex.
The life cycle of Plasmodium is very complex. Sporozoites from the saliva of a biting female mosquito are transmitted to either the blood or the lymphatic system[2] of the recipient. The sporozoites then migrate to the liver and invade hepatocytes. This latent or dormant stage of the Plasmodium sporozoite in the liver is called the hypnozoite.
The development from the hepatic stages to the erythrocytic stages has until very recently been obscure. In 2006[3] it was shown that the parasite buds off the hepatocytes in merosomes containing hundreds or thousands of merozoites. These merosomes have been subsequently shown[4] to lodge in the pulmonary capilaries and to slowly disintergrate there over 48-72 hours releasing merozoites. Erythrocyte invasion is enhanced when blood flow is slow and the cells are tightly packed: both of these conditions are found in the alveolar capilaries.
Within the erythrocytes the merozoite grow first to a ring-shaped form and then to a larger trophozoite form. In the schizont stage, the parasite divides several times to produce new merozoites, which leave the red blood cells and travel within the bloodstream to invade new red blood cells. Most merozoites continue this replicative cycle, but some merozoites differentiate into male or female sexual forms (gametocytes) (also in the blood), which are taken up by the female mosquito.
In the mosquito's midgut, the gametocytes develop into gametes and fertilize each other, forming motile zygotes called ookinetes. The ookinetes penetrate and escape the midgut, then embed themselves onto the exterior of the gut membrane. Here they divide many times to produce large numbers of tiny elongated sporozoites. These sporozoites migrate to the salivary glands of the mosquito where they are injected into the blood of the next host the mosquito bites. The sporozoites move to the liver where they repeat the cycle.
Reactivation of the hypnozoites has been reported for up to 30 years after the initial infection in humans. The factors precipating this reactivation are not known. In the species Plasmodium malariae, Plasmodium ovale and Plasmodium vivax hypnozoites have been shown to occur. Reactivation does not occur in infections with Plasmodium falciparum. It is not known if hypnozoite reactivaction may occur with any of the remaining species that infect humans but this is presumed to be the case.
Evolution
This life cycle is best understood in terms of its evolution.
The Apicomplexia - the phylum to which Plasmodium belongs - are thought to have originated within the Dinoflagellates - a large group of photosynthetic protozoa. It is currently thought that the ancestors of the Apicomplexia were originally prey organisms that evolved the ability to invade the intestinal cells and subsequently lost their photosynthetic ability. Some extant dinoflagelates, however, can invade the bodies of jellyfish and continue to photosynthesize, which is possible because jellyfish bodies are almost transparent. In other organisms with opaque bodies this ability would most likely rapidly be lost.
It is thought that Plasmodium evolved from a parasite spread by the orofaecal route which infected the intestinal wall. At some point this parasite evolved the ability to infect the liver. This pattern is seen in the genus Cryptosporidium to which Plasmodium is distantly related. At some later point this ancestor developed the ability to infect blood cells and to survive and infect mosquitoes. Once mosquito transmission was firmly established the previous orofecal route of transmission was lost.
Current (2007) theory suggests that the genera Plasmodium, Hepatocystis and Haemoproteus evolved from Leukocytozoon species. Parasites of the genus Leukocytozoan infect white blood cells (leukocytes), liver and spleen cells and are transmitted by 'black flies' (Simulium species) - a large genus of flies related to the mosquitoes.
Leukocytes, hepatocytes and most spleen cells actively phagocytose particulate matter making entry into the cell easier for the parasite. The mechanism of entry of Plasmodium species into erythrocytes is still very unclear taking as it does less than 30 seconds. It is not yet known if this mechanism evolved before mosquitoes became the main vectors for transmission of Plasmodium.
Plasmodium evolved about 130 million years ago. This period is coincidental with the rapid spread of the angiosperms (flowering plants). This expansion in the angiosperms is thought to be due to at least one genomic duplication event. It seems probable that the increase in the number of flowers led to an increase in the number of mosquitoes and their contact with vertebrates.
Mosquitoes evolved in what is now South America about 230 million years ago. There are over 3500 species recognised but to date their evolution has not been well worked out so a number of gaps in our knowledge of the evolution of Plasmodium remain.
Presently it seems probable that birds were the first group infected by Plasmodium followed by the reptiles - probably the lizards. At some point primates and rodents became infected. The remaining species infected outside these groups seem likely to be due to relatively recent events.
At the present time (2007) DNA sequences are available from fewer than sixty species and most of these are from species infecting either rodent or primate hosts. The evolution proposed here should be regarded as speculative and subject to revision as data becomes available.
Reproduction
The pattern of alternation of sexual and asexual reproduction which may seem confusing at first is a very common pattern in parasitic species. The evolutionary advantages of this type of life cycle were recognised by Mendel.
Under favourable conditions asexual reproduction is superior to sexual as the parent is well adapted to its environment and its descendents share these genes. Transferring to a new host or in times of stress, sexual reproduction is generally superior as this produces a shuffling of genes which on average at a population level will produce individuals better adapted to the new environment.
Molecular biology
All the species examined to date have 14 chromosomes, one mitochondrion and one plastid. The chromosomes vary from 500 kilobases to 3.5 megabases in length. It is presumed that this is the pattern throughout the genus.
The plastid unlike those found in algae is not photosynthetic. Its function is not known but there is some suggestive evidence that it may be involved in reproduction.
On a molecular level, the parasite damages red blood cells using plasmepsin enzymes - aspartic acid proteases which degrade hemoglobin.
Diagnostic characteristics of the genus Plasmodium
- Forms gamonts in erythrocytes
- Merogony occurs in erythrocytes and in other tissues
- Hemozoin is present
- Vectors are either mosquitos or sandflies
- Vertebrate hosts include mammals, birds and reptiles
Taxonomy
Plasmodium belongs to the family Plasmodiidae (Levine, 1988), order Haemosporidia and phylum Apicomplexia. There are currently 450 recognised species in this order. Many species of this order are undergoing reexamination of their taxonomy with DNA analysis. It seems likely that many of these species will be re assigned after these studies have been completed.[5][6] For this reason the entire order is outlined here.
Notes:
The genera Plasmodium, Fallisia and Saurocytozoon all cause malaria in lizards. All are carried by Dipteria (roughly speaking the flies). Pigment is absent in the Garnia. Non pigmented gametocytes are typically the only forms found in Saurocytozoon: pigmented forms may be found in the leukocytes occasionally. Fallisia produce non pigmented asexual and gametocyte forms in leukocytes and thrombocytes.
Subgenera
The full taxonomic name of a species includes the subgenus but this is often omitted. The full name indicates some features of the morphology and type of host species.
The only two species in the sub genus Laverania are P. falciparum and P. reichenowi.
Species infecting monkeys and apes (the higher primates) with the exceptions of P. falciparum and P. reichenowi are classified in the subgenus Plasmodium.
Parasites infecting other mammals including lower primates (lemurs and others) are classified in the subgenus Vinckeia.
The distinction between P. falciparum and P. reichenowi and the other species infecting higher primates was based on the morphological findings but have since been confirmed by DNA analysis. Vinckeia while previously considered to be something of a taxonomic 'rag bag' has been recently shown - perhaps rather surprisingly - to form a coherent grouping.
The remaining groupings here are based on the morphology of the parasites. Revisions to this system are likely to occur in the future as more species are subject to analysis of their DNA.
The four subgenera Giovannolaia, Haemamoeba, Huffia and Novyella were created by Corradetti et al[7] for the known avian malarial species. A fifth - Bennettinia - was created in 1997 by Valkiunas.[8] The relationships between the subgenera are the matter of current investigation. Martinsen et al 's recent (2006) paper outlines what is currently (2007) known.[9]
P. juxtanucleare is currently (2007) the only known member of the subgenus Bennettinia.
Unlike the mammalian and bird malarias those affecting reptiles have been more difficult to classify. In 1966 Garnham classified those with large schizonts as Sauramoeba, those with small schizonts as Carinamoeba and the single then known species infecting snakes (Plasmodium wenyoni) as Ophidiella.[10] He was aware of the arbitrariness of this system and that it might not prove to be biologically valid. Telford in 1988 used this scheme as the basis for the currently accepted (2007) system.[11]
Classification criteria
Species in the subgenus Bennettinia have the following characteristics:
- Schizonts contain scant cytoplasm, are often round, do not exceed the size of the host nucleus and stick to it.
- Gametocytes while varying in shape tend to be round or oval, do not exceed the size of the nucleus and stick to it.
Species in the subgenus Giovanolaia have the following characteristics:
- Schizonts contain plentiful cytoplasm, are larger than the host cell nucleus and frequently displace it. They are found only in mature erythrocytes.
- Gametocytes are elongated.
- Exoerythrocytic schizogony occurs in the mononuclear phagocyte system.
Species in the subgenus Haemamoeba have the following characteristics:
- Mature schizonts are larger than the host cell nucleus and commonly displace it.
- Gametocytes are large, round, oval or irregular in shape and are substantially larger than the host nucleus.
Species in the subgenus Huffia have the following characteristics:
- Mature schizonts, while varying in shape and size, contain plentiful cytoplasm and are commonly found in immature erthryocytes.
- Gametocytes are elongated.
Species in the subgenus Novyella have the following characteristics:
- Mature schisonts are either smaller than or only slightly larger than the host nucleus. They contain scanty cytoplasm.
- Gametocytes are elongated. Sexual stages in this subgenus resemble those of Haemoproteus.
- Exoerythrocytic schizogony occurs in the mononuclear phagocyte system
Species in the subgenus Carinamoeba have the following characteristics:
- Infect lizards
- Schizonts normally give rise to less than 8 merozoites
Species in the subgenus Sauramoeba have the following characteristics:
- Infect lizards
- Schizonts normally give rise to more than 8 merozoites
Notes
- The erythrocytes of both reptiles and birds retain their nucleus, unlike those of mammals. The reason for the loss of the nucleus in mammalian erythocytes remains unknown.
- The presence of elongated gametocytes in several of the avian subgenera and in Laverania in addition to a number of clinical features suggested that these might be closely related. This is is no longer thought to be the case.
- Plasmodium falciparum (the cause of malignant tertian malaria)
- Plasmodium vivax (the most frequent cause of benign tertian malaria)
- Plasmodium ovale (the other, less frequent, cause of benign tertian malaria)
- Plasmodium malariae (the cause of benign quartan malaria)
- Plasmodium knowlesi
- Plasmodium brasilianum
- Plasmodium cynomolgi
- Plasmodium cynomolgi bastianellii
- Plasmodium inui
- Plasmodium rhodiani
- Plasmodium schweitzi
- Plasmodium semiovale
- Plasmodium simium
The first four listed here are the most common species that infect humans. With the use of the polymerase chain reaction additional species have been and are still being identified that infect humans.
One possible experimental infection has been reported with Plasmodium eylesi. Fever and low grade parasitemia were apparent at 15 days. The volunteer (Dr Bennett) had previously been infected by Plasmodium cynomolgi and the infection was not transferable to a gibbon (P. eylesi 's natural host) so this cannot be regarded as definitive evidence of its ability to infect humans. A second case has been reported that may have been a case of P. eylesi but the author was not certain of the infecting species.[12]
A possible infection with Plasmodium tenue has been reported. [13] This report described a case of malaria in a three year old black girl from Georgia, USA who had never been outside the US. She suffered from both P. falciparum and P. vivax malaria and while forms similar to those described for P. tenue were found in her blood even the author was skeptical about the validity of the diagnosis. Confusingly Plasmodium tenue was proposed in the same year (1914) for a species found in birds. The human species is now considered to be likely to have been a misdiagnosis and the bird species is described on the Plasmodium tenue page.
Notes:
The only known host of P. falciparum are humans; neither is any other host currently known for P. malariae.
P. vivax will infect chimpanzees. Infection tends to be low grade but may be persistent and remain as source of parasites for humans for some time. P. vivax is also known to infect orangutans.[14]
Like P. vivax, P. ovale has been shown to be transmittable to chimpanzees. P. ovale has a unusual distribution pattern being found in Africa, the Philippines and New Guinea. In spite of its admittedly poor transmission to chimpanzees given its discontigous spread, it is suspected that P. ovale may in fact be a zooenosis with an as yet unidentified host. If this is actually the case, the host seems likely to be a primate.
The remaining species capable of infecting humans all have other primate hosts.
Plasmodium shortii and Plasmodium osmaniae are now considered to be junior synonyms of Plasmodium inui
Species no longer recognised as valid
Taxonomy in parasitology until the advent of DNA based methods has always been a problem and revisions in this area are continuing. A number of synonoms have been given for the species infecting humans that are no longer recognised as valid.[15] Since perusal of the older literature may be confusing some of these are listed here...
The species that infect primates other than humans include: P. bouillize, P. brasilianum, P. bucki, P. cercopitheci,P. coatneyi, P. coulangesi, P. cynomolgi, P. eylesi, P. fieldi, P. foleyi, P. fragile, P. girardi, P. georgesi, P. gonderi, P. hylobati, P. inui, P. jefferyi, P. joyeuxi,P. knowlesi, P. lemuris, P. percygarnhami, P. petersi, P. reichenowi, P. rodhaini, P. sandoshami, P. semnopitheci, P. silvaticum, P. simiovale, P. simium, P. uilenbergi, P. vivax and P. youngei.
Host records - Most if not all Plasmodium species infect more than one host: the host records shown here should be regarded as being incomplete.
References
- ↑ Chavatte JM, Chiron F, Chabaud A, Landau I. (2007) Probable speciations by "host-vector 'fidelisation'": 14 species of Plasmodium from Magpies. Parasite 14(1):21-37
- ↑ [http://www.hhmi.org/news/menard20060122.html HHMI Staff (22 January 2006) "Malaria Parasites Develop in Lymph Nodes" HHMI News Howard Hughes Medical Institute
- ↑ Sturm A, Amino R, van de Sand C, Regen T, Retzlaff S, Rennenberg A, Krueger A, Pollok JM, Menard R, Heussler VT. (2006) Manipulation of host hepatocytes by the malaria parasite for delivery into liver sinusoids. Science 313(5791):1287-1290
- ↑ Baer K, Klotz C, Kappe SH, Schnieder T, Frevert U. (2007) Release of Hepatic Plasmodium yoelii Merozoites into the Pulmonary Microvasculature. PLoS Pathog. 3(11):e171
- ↑ Perkins SL, Schall JJ (2002) A molecular phylogeny of malarial parasites recovered from cytochrome b gene sequences. J. Parasitology 88 (5): 972-978
- ↑ Yotoko, K. S. C. and Elisei C. (2006) Malaria parasites (Apicomplexa, Haematozoea) and their relationships with their hosts: is there an evolutionary cost for the specialization? J. Zoo. Syst. Evol. Res. 44 (4) 265
- ↑ Corradetti A., Garnham P. C. C. and Laird M. (1963). New classification of the avian malaria parasites. Parassitologia 5, 1–4
- ↑ Valkiunas, G. (1997). Bird Haemosporidia. Institute of Ecology, Vilnius
- ↑ Martinsen,E. S., Waite J. L. and Schall J. J. (2006) Morphologically defined subgenera of Plasmodium from avian hosts: test of monophyly by phylogenetic analysis of two mitochondrial genes. Parasitology 1-8
- ↑ Garnham P.C.C. (1966) Malaria parasites and other haemospordia. Oxford, Blackwell
- ↑ Telford S. (1988) A contribution to the systematics of the reptilian malaria parasites, family Plasmodiidae (Apicomplexa: Haemosporina). Bulletin of the Florida State Museum Biological Sciences 34, 65-96
- ↑ Tsukamoto M. (1977) An imported human malarial case characterized by severe multiple infections of the red blood cells. Ann. Trop. Med. Parasit. 19(2)95-104
- ↑ Russel P.F. (1928) Plasmodium tenue (Stephens): A review of the literature and a case report. Am. J. Trop. Med. s1-8(5) 449-479
- ↑ Reid MJ. (2006) Transmission of Human and Macaque Plasmodium spp. to Ex-Captive Orangutans in Kalimantan, Indonesia. Emerg Infect Dis. 12(12):1902-1908
- ↑ Coatney G. R. and Roudabush R. L. (1936) A catalog and host-index of the genus Plasmodium. J. Parasitol. 22 (4) 338-353
Template:SIB ar:متصورة ca:Plasmodi de:Plasmodium eo:Plasmodio hr:Plasmodium id:Plasmodium it:Plasmodium he:Plasmodium lt:Plazmodis ms:Plasmodium sr:Plasmodium sv:Plasmodium (släkte) ta:பிளாஸ்மோடியம் th:พลาสโมเดียม
Natural History, Complications & Prognosis
Diagnosis
{{#ask:Used To Diagnose::Malaria |?Sort Order |format=list |headers=hide |link=none |sep= | |template=MedicalTestQuery |sort=Sort Order }}
Treatment
{{#ask:Used To Treat::Malaria |?Sort Order |format=list |headers=hide |link=none |sep= | |template=MedicalTreatmentQuery |sort=Sort Order }} {{#ask:Prevents::Malaria |?Sort Order |intro= | |format=list |headers=hide |link=none |sep= | |template=MedicalTreatmentQuery2 |sort=Sort Order }}
References
External links
- National Geographic July 2007 Issue on Malaria
- WHO site on malaria
- Template:McGrawHillAnimation
- Johns Hopkins Malariology Open Courseware
- www.malariacontrol.net distributed computing project for the fight against malaria
- United States Centers for Disease Control - Malaria information pages
- Medline Plus - Malaria
- Interview with Dr Andrew Speilman, Harvard malaria specialist
- Malaria Consortium website
- GlobalHealthFacts.org Malaria Cases and Deaths by Country
- Survey article: History of malaria around the North Sea
- DriveAgainstMalaria.org, "World's longest journey to fight the biggest killer of children"
- Malaria on JHSPH OpenCourseWare
- Malaria Foundation International
- Malaria Atlas Project
- UNITAID, International Facility for the Purchase of Drugs (Wikipedia Article)
- BBC - Hopes of Malaria Vaccine by 2010 15 October 2004
- BBC - Science shows how malaria hides 8 April 2005
- History of discoveries in malaria
- Malaria. The UNICEF-UNDP-World Bank-WHO Special Programme for Research and Training in Tropical Diseases
- Malaria Vaccine Initiative
- "Vaccines for Development" - Blog on vaccine research and production for developing countries
- Medicines for Malaria Venture
- Malaria and Mosquitos - questions and answers
- Hisnets - Fighting Malaria: One Net At A Time
- Call for Increased Production of Long-Lasting Insecticidal Nets as Part of the U.N. Millenium Campaign
- Burden of Malaria, BBC pictures relating to malaria in northern Uganda
- Malaria: Cooperation among Parasite, Vector, and Host (Animation)
Template:Link FA Template:Protozoal diseases
Template:Link FA Template:Link FA Template:Link FA af:Malaria ar:ملاريا zh-min-nan:Ma-lá-lí-á bs:Malarija bg:Малария ca:Malària cs:Malárie da:Malaria de:Malaria el:Ελονοσία eo:Malario eu:Malaria gl:Malaria ko:말라리아 hi:शीतज्वर hr:Malarija id:Malaria ia:Malaria it:Malaria he:מלריה ka:მალარია hu:Malária mt:Malarja ms:Malaria nl:Malaria no:Malaria om:Malaria ps:ملاريا qu:Chukchu simple:Malaria sk:Malária sl:Malarija sr:Маларија sh:Malarija su:Malaria fi:Malaria sv:Malaria ta:மலேரியா te:మలేరియా th:มาลาเรีย uk:Малярія
- Pages with reference errors
- CS1 maint: PMC format
- CS1 maint: Explicit use of et al.
- CS1 maint: Multiple names: authors list
- CS1 errors: dates
- Apicomplexa
- Insect-borne diseases
- Malaria
- Emergency medicine
- Parasitic diseases
- Tropical disease
- Deaths from malaria
- Disease
- Parasites
- Infectious disease
- Mature chapter
- Overview complete