Keratin
WikiDoc Resources for Keratin |
Articles |
---|
Most recent articles on Keratin |
Media |
Evidence Based Medicine |
Clinical Trials |
Ongoing Trials on Keratin at Clinical Trials.gov Clinical Trials on Keratin at Google
|
Guidelines / Policies / Govt |
US National Guidelines Clearinghouse on Keratin
|
Books |
News |
Commentary |
Definitions |
Patient Resources / Community |
Directions to Hospitals Treating Keratin Risk calculators and risk factors for Keratin
|
Healthcare Provider Resources |
Causes & Risk Factors for Keratin |
Continuing Medical Education (CME) |
International |
|
Business |
Experimental / Informatics |
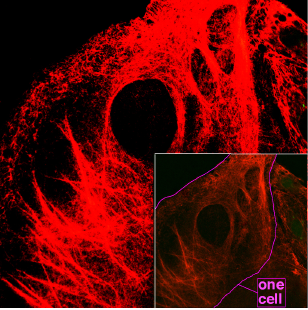
Keratins are a family of fibrous structural proteins; tough and insoluble, they form the hard but nonmineralized structures found in reptiles, birds, amphibians and mammals. They are rivaled as biological materials in toughness only by chitin.
There are various types of keratins within a single animal.
Variety of animal uses
Keratins are the main constituent of structures that grow from the skin:
- the α-keratins in the hair (including wool), horns, nails, claws and hooves of mammals[verification needed]
- the harder β-keratins found in nails and in the scales and claws of reptiles, their shells (chelonians, such as tortoise, turtle, terrapin), and in the feathers, beaks, and claws of birds.[verification needed] (These keratins are formed primarily in beta sheets. However, beta sheets are also found in α-keratins.)[1]
Arthropods such as crustaceans often have parts of their armor or exoskeleton made of keratin, sometimes in combination with chitin.
The baleen plates of filter-feeding whales are made of keratin.
They can be integrated in the chitinophosphatic material that makes up the shell and setae in many brachiopods.
Keratins are also found in the gastrointestinal tracts of many animals, including roundworms (who also have an outer layer made of keratin).
Although it is now difficult to be certain, the scales, claws, some protective armour and the beaks of dinosaurs would, almost certainly, have been composed of a type of keratin.
In Crossopterygian fish, the outer layer of cosmoid scales was keratin.
Cornification
In mammals there are soft epithelial keratins, the cytokeratins, and harder hair keratins. As certain skin cells differentiate and become cornified, pre-keratin polypeptides are incorporated into intermediate filaments. Eventually the nucleus and cytoplasmic organelles disappear, metabolism ceases and cells undergo a programmed death as they become fully keratinized.
Cells in the epidermis contain a structural matrix of keratin which makes this outermost layer of the skin almost waterproof, and along with collagen and elastin, gives skin its strength. Rubbing and pressure cause keratin to proliferate with the formation of protective calluses — useful for athletes and on the fingertips of musicians who play stringed instruments. Keratinized epidermal cells are constantly shed and replaced (see dandruff).
These hard, integumentary structures are formed by intercellular cementing of fibers formed from the dead, cornified cells generated by specialized beds deep within the skin. Hair grows continuously and feathers moult and regenerate. The constituent proteins may be phylogenetically homologous but differ somewhat in chemical structure and supermolecular organization. The evolutionary relationships are complex and only partially known. Multiple genes have been identified for the β-keratins in feathers, and this is probably characteristic of all keratins.
Molecular biology and biochemistry
The properties which make structural proteins like keratins useful depend on their supermolecular aggregation. These depend on the properties of the individual polypeptide strands, which depend in turn on their amino acid composition and sequence. The α-helix and β-sheet motifs, and disulfide bridges, are crucial to the conformations of globular, functional proteins like enzymes, many of which operate semi-independently, but they take on a completely dominant role in the architecture and aggregation of keratins.
Glycine and alanine
Keratins contain a high proportion of the smallest of the 20 amino acids, glycine, whose "side group" is a single hydrogen atom; also the next smallest, alanine, with a small and noncharged methyl group. In the case of β-sheets, this allows sterically-unhindered hydrogen bonding between the amino and carboxyl groups of peptide bonds on adjacent protein chains, facilitating their close alignment and strong binding. Fibrous keratin molecules can twist around each other to form helical intermediate filaments.
Limited interior space is the reason why the triple helix of the (unrelated) structural protein collagen, found in skin, cartilage and bone, likewise has a high percentage of glycine. The connective tissue protein elastin also has a high percentage of both glycine and alanine. Silk fibroin, considered a β-keratin, can have these two as 75–80% of the total, with 10–15% serine, with the rest having bulky side groups. The chains are antiparallel, with an alternating C → N orientation.[1] A preponderance of amino acids with small, nonreactive side groups is characteristic of structural proteins, for which H-bonded close packing is more important than chemical specificity.
Disulfide bridges
In addition to intra- and intermolecular hydrogen bonds, keratins have large amounts of the sulfur-containing amino acid cysteine, required for the disulfide bridges that confer additional strength and rigidity by permanent, thermally-stable crosslinking—a role sulfur bridges also play in vulcanized rubber. Human hair is approximately 14% cysteine. The pungent smells of burning hair and rubber are due to the sulfur compounds formed. Extensive disulfide bonding contributes to the insolubility of keratins, except in dissociating or reducing agents.
The more flexible and elastic keratins of hair have fewer interchain disulfide bridges than the keratins in mammalian fingernails, hooves and claws (homologous structures), which are harder and more like their analogs in other vertebrate classes. Hair and other α-keratins consist of α-helically-coiled single protein strands (with regular intra-chain H-bonding), which are then further twisted into superhelical ropes that may be further coiled. The β-keratins of reptiles and birds have β-pleated sheets twisted together, then stabilized and hardened by disulfide bridges.
Silk
The silk fibroins produced by insects and spiders are often classified as keratins, though it is unclear whether they are phylogenetically related to vertebrate keratins.
Silk found in insect pupae, and in spider webs and egg casings, also has twisted β-pleated sheets incorporated into fibers wound into larger supermolecular aggregates. The structure of the spinnerets on spiders’ tails, and the contributions of their interior glands, provide remarkable control of fast extrusion. Spider silk is typically about 1 to 2 micrometres (µm) thick, compared with about 60 µm for human hair, and more for some mammals. (Hair, or fur, occurs only in mammals.) The biologically and commercially useful properties of silk fibers depend on the organization of multiple adjacent protein chains into hard, crystalline regions of varying size, alternating with flexible, amorphous regions where the chains are randomly coiled.[2] A somewhat analogous situation occurs with synthetic polymers such as nylon, developed as a silk substitute. Silk from the hornet cocoon contains doublets about 10 µm across, with cores and coating, and may be arranged in up to 10 layers; also in plaques of variable shape. Adult hornets also use silk as a glue, as do spiders.
Pairing
A (neutral-basic) | B (acidic) | Occurrence |
keratin 1, keratin 2 | keratin 9, keratin 10 | stratum corneum, keratinocytes |
keratin 3 | keratin 12 | cornea |
keratin 4 | keratin 13 | stratified epithelium |
keratin 5 | keratin 14, keratin 15 | stratified epithelium |
keratin 6 | keratin 16, keratin 17 | squamous epithelium |
keratin 7 | keratin 19 | ductal epithelia |
keratin 8 | keratin 18, keratin 20 | simple epithelium |
Clinical significance
Some infectious fungi, such as those which cause athlete's foot, ringworm or the Batrachochytrium dendrobatidis (Chytrid fungus) which is killing amphibians all over the world, feed on keratin.
Diseases caused by mutations in the keratin genes include
- Epidermolysis bullosa simplex
- Ichthyosis bullosa of Siemens
- Epidermolytic hyperkeratosis
- Steatocystoma multiplex
See also
Additional images
-
Keratin (high molecular weight) in bile duct cell and oval cells of mouse liver.
References
- ↑ Kreplak L, Doucet J, Dumas P, Briki F (2004). "New aspects of the alpha-helix to beta-sheet transition in stretched hard alpha-keratin fibers". Biophys J. 87 (1): 640–7. doi:10.1529/biophysj.103.036749. PMID 15240497.
- ↑ Spiders - Silk structure
External links
- Composition and β-sheet structure of silk
- Spider silk fiber structure
- Hair-Science.com's entry on the miroscopic elements of hair
- keratin antibody review
am:ኬራቲን bg:Кератин ca:Ceratina cs:Keratin da:Keratin de:Keratin eo:Keratino fi:Keratiini gl:Queratina he:קרטין hr:Keratin it:Cheratina lt:Keratinas nl:Keratine no:Keratin oc:Queratina simple:Keratin su:Keratin sv:Keratin ta:நகமியம் ur:قرنین