Thalassemia pathophysiology
Thalassemia Microchapters |
Diagnosis |
---|
Treatment |
Case Studies |
Thalassemia pathophysiology On the Web |
American Roentgen Ray Society Images of Thalassemia pathophysiology |
Risk calculators and risk factors for Thalassemia pathophysiology |
Editor-In-Chief: C. Michael Gibson, M.S., M.D. [1] Shyam Patel [2] Neel Patel, M.B.B.S[3]
Overview
The pathophysiology of alpha- and beta-thalassemia involves abnormal production of globin chains. Alpha- and beta-thalassemias are both monogenic disorders, meaning that defects in one gene result in the disease. The pathogenesis of thalassemias can involve a various of mutational events, such as deletions, insertions, or point mutations (substitutions). The altered genetic sequence results in a gene product (protein) that is nonfunctional or dysfunctional, such that the new globin chain cannot effective deliver oxygen to peripheral tissues. The number of alleles that are lost on each globin-cluster determines the severity of the disease. Regardless of the type of mutation, the thalassemias are inherited in a Mendelian autosomal recessive fashion.
Pathophysiology
In order to understand the pathophysiology of thalassemia, one must first understand the normal physiology of globin gene synthesis and hemoglobin production.
Normal Physiology
- Normal adult hemoglobin: Normal adult hemoglobin is hemoglobin A (HbA), which consists of 2 alpha-globin chains and 2 beta-globin chains.[1] This forms a tetramer of alpha2-beta2. Normal adults also harbor a small component of hemoglobin A2 (HbA2), which consists of 2 alpha-globin chains and 2 delta-globin chains. This forms a tetramer of alpha2-delta2. In the center of each globin chain tetramer is a heme molecule, which binds to iron. Thus the molecular ratio of globin to heme to iron is 4:1:1. Oxygen binds to heme, and heme thus transports iron throughout the body. Normal adults do not have any circulating hemoglobin containing beta-4 tetramers, gamma-4 tetramers, or sickled hemoglobin.[1]
- Normal alpha-globin gene cluster: The alpha-globin gene cluster is near the telomere of the short arm of chromosome 16 (16p13.3). The globin genes located on the alpha-globin cluster on chromosome 16 are transcribed from the 5' to 3' direction of the chromosome. The first globin gene transcribed from the alpha-globin cluster is zeta2-globin, then zeta1-globin, then alpha2-globin, then alpha1-globin (from telomere to centromere).[2] Upstream of the alpha-globin gene cluster are four conserved noncoding DNA sequences that are thought to serve a regulatory role in alpha-globin chain synthesis.
- Normal beta-globin gene cluster: The genes located on the beta-globin cluster on chromosome 11 are transcribed from the 5' to 3' direction of the chromosome. The first globin that is transcribed is epsilon-globin. This is followed by transcription of the G-gamma-globin, then A-gamma-globin, then delta-globin, then the normal adult beta-globin [2]
- Hemoglobin tetramer synthesis: In order to produce a hemoglobin molecule, two proteins (gene products) from the alpha-globin gene cluster must pair with two proteins (gene products) from the beta-globin gene cluster. A variety of tetrameric combinations can result. The order of synthesis of hemoglobin tetramers during human development recapitulates the location of the globin genes on their respective chromosomes. For example, the genes that are transcribed first are the genes that constitute embryonic and fetal hemoglobin. The genes that are transcribed last are the genes that constitute mature adult hemoglobin.
- Compensatory globin production: If there is defective alpha-globin chain production, alpha-thalassemia results, with excess of beta-globin chains. If there is defective beta-globin chain production, beta-thalassemia results, with excess of alpha-globin chains.[1]
Pathophysiology of alpha-thalassemias
The alpha-thalassemias involve defects in the genes HBA1 (Online Mendelian Inheritance in Man (OMIM) 141800) and HBA2 (Online Mendelian Inheritance in Man (OMIM) 141850), inherited in a Mendelian autosomal recessive fashion. It is also related to the deletion of the 16p chromosome, where the alpha-globin gene cluster resides. Alpha-thalassemias result in decreased alpha-globin production, therefore fewer alpha-globin chains are produced, resulting in an excess of beta-chains in adults and excess gamma-globin chains in newborns. The excess beta-chains form unstable tetramers (called Hemoglobin H or HbH of 4 beta chains) which have abnormal oxygen dissociation curves. In the presence of excess beta-globin chains, red blood cell membrane instability develops and hemolysis ensues.
Any given individual has four alpha-globin alleles. Two of these are maternal in origin and two of these are paternal in origin. The severity of the α thalassemias is correlated with the number of affected α globin loci: the greater the number of affected loci, the more severe will be the manifestations of the disease.
- If one of the four alpha-globin loci is affected, there is minimal effect. Three alpha-globin loci are enough to permit normal hemoglobin production, and there is no anemia or hypochromia in these people. They have been called silent carriers.
- If two of the four alpha-globin loci are affected, the condition is called alpha thalassemia trait. Two alpha-globin loci permit nearly normal erythropoiesis, but there is a mild microcytic hypochromic anemia. The disease in this form can be mistaken for iron-deficiency anemia and treated inappropriately with iron. Alpha-thalassemia trait can exist in two forms: one form, associated with Asians, involves cis deletion of two alpha loci on the same chromosome; the other, associated with Blacks, involves trans deletion of alpha loci on different (homologous) chromosomes.
- If three of the four alpha-globin loci are affected, the condition is called Hemoglobin H disease. Hemoglobin H is a tetrameric beta-globin protein complex. This has a higher affinity for oxygen than normal hemoglobin, resulting in poor oxygen delivery to tissues. There is a microcytic hypochromic anemia with target cells and Heinz bodies (precipitated HbH) on the peripheral blood smear, as well as splenomegaly. The disease may first be noticed in childhood or in early adult life, when the anemia and splenomegaly are noted.
- If all four of the alpha-globin loci are affected, the fetus cannot live once outside the uterus and may not survive gestation: most such infants are dead at birth with hydrops fetalis, and those who are born alive die shortly after birth. They are edematous and have little circulating hemoglobin, and the hemoglobin that is present is all tetrameric gamma-globin chains (hemoglobin Barts).
Pathophysiology of beta-thalassemias
Beta-thalassemias are caused by defects in the HBB gene (beta-globin chain) on chromosome 11 (Online Mendelian Inheritance in Man (OMIM) 141900), also inherited in an Mendelian autosomal recessive fashion. There are over 300 known mutations that occur in beta-globin that can cause thalassemia.[3] The severity of the disease, need for transfusion and morbidity depends on the nature of the mutation and imbalance between alpha and beta subunits.[4] Mutations are characterized as B0 if they prevent any formation of beta-globin chains; they are characterized as B+ if they allow some beta-globin chain formation to occur. In either case there is a relative excess of alpha-globin chains, but these do not form tetramers: rather, they bind to the red blood cell membranes, producing membrane damage, and at high concentrations they form toxic aggregates. In beta thalassemia, red cell precursors can detoxify and tolerate a moderate amount of free alpha globin, that is stabilized by alpha hemoglobin stabilizing protein and eliminated by ubiquitin proteasome system and autophagy. The synthesis of gamma globin gene and HbF can reduce the degree of imbalance between alpha and beta chains. BCL11A(a multi zinc finger transcription regulator) plays a key role in switch from fetal to adult hemoglobin and silencing of the fetal hemoglobin. Genetic variations in BCL11A causing persistent HbF production reduce the clinical severity of beta thalassemia.
Any given individual has two beta-globin globin alleles, one inherited from the mother and one inherited from the father.
- If only one beta-globin allele bears a mutation, the disease is called beta-thalassemia minor (or sometimes called beta-thalassemia trait). This is a mild microcytic anemia. In most cases beta-thalassemia minor is asymptomatic, and many affected people are unaware of the disorder. Detection usually involves measuring the mean corpuscular volume (size of red blood cells) and noticing a slightly decreased mean volume than normal. The patient will have an increased fraction of Hemoglobin A2 (>2.5%) and a decreased fraction of Hemoglobin A (<97.5%).
- If both alleles have thalassemia mutations, the disease is called beta-thalassemia major or Cooley's anemia. This is a severe microcytic, hypochromic anemia. Untreated, this progresses to death before age twenty. Treatment consists of periodic blood transfusion; splenectomy if splenomegaly is present, and treatment of transfusion-caused iron overload. Cure is possible by bone marrow transplantation.
- Thalassemia intermedia is a condition intermediate between the major and minor forms. Affected individuals can often manage a normal life but may need occasional transfusions e.g. at times of illness or pregnancy, depending on the severity of their anemia.
The genetic mutations present in beta-thalassemias are very diverse, and a number of different mutations can cause reduced or absent beta-globin synthesis. Two major groups of mutations can be distinguished:
- Nondeletion forms: These defects generally involve a single base substitution or small deletion or inserts near or upstream of the beta-globin gene. Most commonly, mutations occur in the promoter regions preceding the beta-globin genes. Less often, abnormal splice variants are believed to contribute to the disease. Most beta-thalassemia mutations are point mutations, in which one nucleotide becomes substituted for another nucleotide.
- Deletion forms: Deletions of different sizes involving the beta-globin gene produce different syndromes such as (B0 thalassemia) or hereditary persistence of fetal hemoglobin syndromes.
Pathophysiology of delta-thalassemias
In addition to alpha-globin and beta-globin chains being present in hemoglobin, about 3% of adult hemoglobin is made of alpha-globin and delta-globin tetramers (hemoglobin A2) (HbA2).[5]
- Mutation: Just as with beta-thalassemia, mutations can occur which affect the ability of this gene to produce delta chains. A mutation that prevents formation of any delta chains is termed a delta0 mutation, whereas one that decreases but does not eliminate production of delta chain is termed a delta+ mutation. When one inherits two delta0 mutations in a Mendelian fashion, no HbA2 (alpha2-delta2) can be formed.
- Clinical manifestations: Hematologically, however, this is innocuous because only 2-3% of normal adult hemoglobin is HbA2. The individual will have normal hematological parameters (erythrocyte count, total hemoglobin, mean corpuscular volume, red cell distribution width). Individuals who inherit only one delta-thalassemia mutation will have a decreased HbA2, but also no hematological consequences. In some cases, co-inheritance of delta-globin and beta-globin mutations can cause elevated fetal hemoglobin.[6]
- Distinguishing delta-thalassemia from beta-thalassemia: The importance of recognizing the existence of delta thalassemia is seen best in cases where it may mask the diagnosis of beta-thalassemia trait. In beta-thalassemia, there is an increase in HbA2, typically in the range of 4-6% (normal is 2-3%). However, the co-existence of a delta-thalassemia mutation will decrease the value of the HbA2 into the normal range, thereby obscurring the diagnosis of beta-thalassemia trait. This can be important in genetic counseling, because a child who is the product of parents each of whom has B0 thalassemia trait has a one in four chance of having beta-thalassemia major, or Cooley's anemia.
In combination with other hemoglobinopathies
Thalassemia can co-exist with other hemoglobinopathies. The most common of these are:
- Hemoglobin E/thalassemia: common in Cambodia, Thailand, and parts of India; clinically similar to β thalassemia major or thalassemia intermedia.
- Hemoglobin S/thalassemia, common in African and Mediterranean populations; clinically similar to sickle cell anemia, with the additional feature of splenomegaly
- Hemoglobin C/thalassemia: common in Mediterranean and African populations, hemoglobin C/βo thalassemia causes a moderately severe hemolytic anemia with splenomegaly; hemoglobin C/β+ thalassemia produces a milder disease.
Genetics
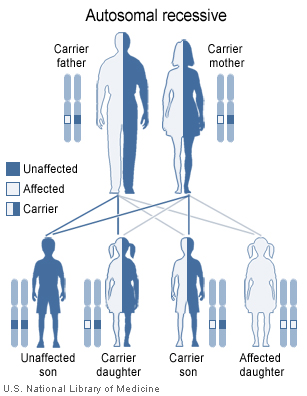
alpha- and beta-thalassemias are often inherited in an autosomal recessive fashion although this is not always the case. Reports of dominantly inherited alpha- and beta-thalassemias have been reported the first of which was in an Irish family who had a two deletions of 4 and 11 bp in exon 3 interrupted by an insertion of 5 bp in the β-globin gene. For the autosomal recessive forms of the disease both parents must be carriers in order for a child to be affected. If both parents carry a hemoglobinopathy trait, there is a 25% chance with each pregnancy for an affected child. Genetic counseling and genetic testing is recommended for families that carry a thalassemia trait.
There are an estimated 60-80 million people in the world who carry the beta thalassemia trait alone. This is a very rough estimate and the actual number of thalassemia Major patients is unknown due to the prevalence of thalassemia in less developed countries in the Middle East and Asia. Countries such as India, Pakistan and Iran are seeing a large increase of thalassemia patients due to lack of genetic counseling and screening. There is growing concern that thalassemia may become a very serious problem in the next 50 years, one that will burden the world's blood bank supplies and the health system in general. There are an estimated 1,000 people living with thalassemia major in the United States and an unknown number of carriers. Because of the prevalence of the disease in countries with little knowledge of thalassemia, access to proper treatment and diagnosis can be difficult.
Benefits of Thalassemia
Being a carrier of the disease may confer a degree of protection against malaria, and is quite common among people from Italian or Greek origin, and also in some African and Indian regions. This is probably by making the red blood cells more susceptible to the less lethal species Plasmodium vivax, simultaneously making the host RBC environment unsuitable for the merozoites of the lethal strain Plasmodium falciparum. This is believed to be a selective survival advantage for patients with the various thalassemia traits. In that respect it resembles another genetic disorder, sickle-cell disease.
Epidemiological evidence from Kenya suggests another reason: protection against severe anemia may be the advantage.[7].
People diagnosed with heterozygous (carrier) Beta-Thalassemia have some protection against coronary heart disease.[8]
References
- ↑ 1.0 1.1 1.2 Harteveld CL, Higgs DR (2010). "Alpha-thalassaemia". Orphanet J Rare Dis. 5: 13. doi:10.1186/1750-1172-5-13. PMC 2887799. PMID 20507641.
- ↑ 2.0 2.1 Higgs DR (2013). "The molecular basis of α-thalassemia". Cold Spring Harb Perspect Med. 3 (1): a011718. doi:10.1101/cshperspect.a011718. PMC 3530043. PMID 23284078.
- ↑ Finotti A, Breda L, Lederer CW, Bianchi N, Zuccato C, Kleanthous M; et al. (2015). "Recent trends in the gene therapy of β-thalassemia". J Blood Med. 6: 69–85. doi:10.2147/JBM.S46256. PMC 4342371. PMID 25737641.
- ↑ Saki N, Abroun S, Soleimani M, Kavianpour M, Shahjahani M, Mohammadi-Asl J; et al. (2016). "MicroRNA Expression in β-Thalassemia and Sickle Cell Disease: A Role in The Induction of Fetal Hemoglobin". Cell J. 17 (4): 583–92. PMC 4746408. PMID 26862517.
- ↑ Kimura A, Matsunaga E, Ohta Y, Fujiyoshi T, Matsuo T, Nakamura T; et al. (1982). "Structure of cloned delta-globin genes from a normal subject and a patient with delta-thalassemia; sequence polymorphisms found in the delta-globin gene region of Japanese individuals". Nucleic Acids Res. 10 (19): 5725–32. PMC 320924. PMID 6292847.
- ↑ Verma S, Bhargava M, Mittal S, Gupta R (2013). "Homozygous delta-beta Thalassemia in a Child: a Rare Cause of Elevated Fetal Hemoglobin". Iran J Ped Hematol Oncol. 3 (1): 222–7. PMC 3915439. PMID 24575268.
- ↑ Wambua S, Mwangi TW, Kortok M, Uyoga SM, Macharia AW, Mwacharo JK, Weatherall DJ, Snow RW, Marsh K, Williams TN (2006). "The effect of α+-Thalassaemia on the Incidence of Malaria and other diseases in children living on the coast of Kenya". PLoS Med 3(5): e158.
- ↑ Tassiopoulos S,Deftereos S,Konstantopoulos K,Farmakis D,Tsironi M,Kyriakidis M,Aessopos A. (2005). "Does heterozygous beta-thalassemia confer a protection against coronary artery disease?". Ann N Y Acad Sci. 1053: 467&ndash, 70.
- ↑ Taher AT, Musallam KM, Cappellini MD (2021). "β-Thalassemias". N Engl J Med. 384 (8): 727–743. doi:10.1056/NEJMra2021838. PMID 33626255 Check
|pmid=
value (help).